
TED日本語
TED Talks(英語 日本語字幕付き動画)
TED日本語 - シェリル・ハヤシ: クモの糸の壮麗さ
TED Talks
クモの糸の壮麗さ
The magnificence of spider silk
シェリル・ハヤシ
Cheryl Hayashi
内容
シェリル・ハヤシはクモの糸を研究しています。自然界に存在する高性能な素材のひとつです。単一のクモの種でも、最大7種類の非常に異なる質の糸を作る能力があります。どうやって作りだすのか シェリル・ハヤシが DNA の説明をし、超強力で超柔軟な素材がどんなに興奮するもので、アイデアをくれるものか教えてくれます
字幕
SCRIPT
Script
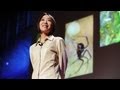
I'm here to spread the word about the magnificence of spiders and how much we can learn from them. Spiders are truly global citizens. You can find spiders in nearly every terrestrial habitat. This red dot marks the Great Basin of North America, and I'm involved with an alpine biodiversity project there with some collaborators. Here's one of our field sites, and just to give you a sense of perspective, this little blue smudge here, that's one of my collaborators. This is a rugged and barren landscape, yet there are quite a few spiders here. Turning rocks over revealed this crab spider grappling with a beetle.
Spiders are not just everywhere, but they're extremely diverse. There are over 40,000 described species of spiders. To put that number into perspective, here's a graph comparing the 40,000 species of spiders to the 400 species of primates. There are two orders of magnitude more spiders than primates. Spiders are also extremely old. On the bottom here, this is the geologic timescale, and the numbers on it indicate millions of years from the present, so the zero here, that would be today. So what this figure shows is that spiders date back to almost 380 million years. To put that into perspective, this red vertical bar here marks the divergence time of humans from chimpanzees, a mere seven million years ago.
All spiders make silk at some point in their life. Most spiders use copious amounts of silk, and silk is essential to their survival and reproduction. Even fossil spiders can make silk, as we can see from this impression of a spinneret on this fossil spider. So this means that both spiders and spider silk have been around for 380 million years. It doesn't take long from working with spiders to start noticing how essential silk is to just about every aspect of their life. Spiders use silk for many purposes, including the trailing safety dragline, wrapping eggs for reproduction, protective retreats and catching prey.
There are many kinds of spider silk. For example, this garden spider can make seven different kinds of silks. When you look at this orb web, you're actually seeing many types of silk fibers. The frame and radii of this web is made up of one type of silk, while the capture spiral is a composite of two different silks: the filament and the sticky droplet. How does an individual spider make so many kinds of silk? To answer that, you have to look a lot closer at the spinneret region of a spider. So silk comes out of the spinnerets, and for those of us spider silk biologists, this is what we call the "business end" of the spider. (Laughter) We spend long days ... Hey! Don't laugh. That's my life. (Laughter) We spend long days and nights staring at this part of the spider. And this is what we see. You can see multiple fibers coming out of the spinnerets, because each spinneret has many spigots on it. Each of these silk fibers exits from the spigot, and if you were to trace the fiber back into the spider, what you would find is that each spigot connects to its own individual silk gland. A silk gland kind of looks like a sac with a lot of silk proteins stuck inside. So if you ever have the opportunity to dissect an orb-web-weaving spider, and I hope you do, what you would find is a bounty of beautiful, translucent silk glands.
Inside each spider, there are hundreds of silk glands, sometimes thousands. These can be grouped into seven categories. They differ by size, shape, and sometimes even color. In an orb-web-weaving spider, you can find seven types of silk glands, and what I have depicted here in this picture, let's start at the one o'clock position, there's tubuliform silk glands, which are used to make the outer silk of an egg sac. There's the aggregate and flagelliform silk glands which combine to make the sticky capture spiral of an orb web. Pyriform silk glands make the attachment cement -- that's the silk that's used to adhere silk lines to a substrate. There's also aciniform silk, which is used to wrap prey. Minor ampullate silk is used in web construction. And the most studied silk line of them all: major ampullate silk. This is the silk that's used to make the frame and radii of an orb web, and also the safety trailing dragline.
But what, exactly, is spider silk? Spider silk is almost entirely protein. Nearly all of these proteins can be explained by a single gene family, so this means that the diversity of silk types we see today is encoded by one gene family, so presumably the original spider ancestor made one kind of silk, and over the last 380 million years, that one silk gene has duplicated and then diverged, specialized, over and over and over again, to get the large variety of flavors of spider silks that we have today. There are several features that all these silks have in common. They all have a common design, such as they're all very long -- they're sort of outlandishly long compared to other proteins. They're very repetitive, and they're very rich in the amino acids glycine and alanine. To give you an idea of what a spider silk protein looks like, this is a dragline silk protein, it's just a portion of it, from the black widow spider. This is the kind of sequence that I love looking at day and night. (Laughter)
So what you're seeing here is the one letter abbreviation for amino acids, and I've colored in the glycines with green, and the alanines in red, and so you can see it's just a lot of G's and A's. You can also see that there's a lot of short sequence motifs that repeat over and over and over again, so for example there's a lot of what we call polyalanines, or iterated A's, AAAAA. There's GGQ. There's GGY. You can think of these short motifs that repeat over and over again as words, and these words occur in sentences. So for example this would be one sentence, and you would get this sort of green region and the red polyalanine, that repeats over and over and over again, and you can have that hundreds and hundreds and hundreds of times within an individual silk molecule.
Silks made by the same spider can have dramatically different repeat sequences. At the top of the screen, you're seeing the repeat unit from the dragline silk of a garden argiope spider. It's short. And on the bottom, this is the repeat sequence for the egg case, or tubuliform silk protein, for the exact same spider. And you can see how dramatically different these silk proteins are -- so this is sort of the beauty of the diversification of the spider silk gene family. You can see that the repeat units differ in length. They also differ in sequence. So I've colored in the glycines again in green, alanine in red, and the serines, the letter S, in purple. And you can see that the top repeat unit can be explained almost entirely by green and red, and the bottom repeat unit has a substantial amount of purple. What silk biologists do is we try to relate these sequences, these amino acid sequences, to the mechanical properties of the silk fibers.
Now, it's really convenient that spiders use their silk completely outside their body. This makes testing spider silk really, really easy to do in the laboratory, because we're actually, you know, testing it in air that's exactly the environment that spiders are using their silk proteins. So this makes quantifying silk properties by methods such as tensile testing, which is basically, you know, tugging on one end of the fiber, very amenable. Here are stress-strain curves generated by tensile testing five fibers made by the same spider. So what you can see here is that the five fibers have different behaviors. Specifically, if you look on the vertical axis, that's stress. If you look at the maximum stress value for each of these fibers, you can see that there's a lot of variation, and in fact dragline, or major ampullate silk, is the strongest of these fibers. We think that's because the dragline silk, which is used to make the frame and radii for a web, needs to be very strong.
On the other hand, if you were to look at strain -- this is how much a fiber can be extended -- if you look at the maximum value here, again, there's a lot of variation and the clear winner is flagelliform, or the capture spiral filament. In fact, this flagelliform fiber can actually stretch over twice its original length. So silk fibers vary in their strength and also their extensibility. In the case of the capture spiral, it needs to be so stretchy to absorb the impact of flying prey. If it wasn't able to stretch so much, then basically when an insect hit the web, it would just trampoline right off of it. So if the web was made entirely out of dragline silk, an insect is very likely to just bounce right off. But by having really, really stretchy capture spiral silk, the web is actually able to absorb the impact of that intercepted prey.
There's quite a bit of variation within the fibers that an individual spider can make. We call that the tool kit of a spider. That's what the spider has to interact with their environment. But how about variation among spider species, so looking at one type of silk and looking at different species of spiders? This is an area that's largely unexplored but here's a little bit of data I can show you. This is the comparison of the toughness of the dragline spilk spun by 21 species of spiders. Some of them are orb-weaving spiders and some of them are non-orb-weaving spiders. It's been hypothesized that orb-weaving spiders, like this argiope here, should have the toughest dragline silks because they must intercept flying prey. What you see here on this toughness graph is the higher the black dot is on the graph, the higher the toughness.
The 21 species are indicated here by this phylogeny, this evolutionary tree, that shows their genetic relationships, and I've colored in yellow the orb-web-weaving spiders. If you look right here at the two red arrows, they point to the toughness values for the draglines of nephila clavipes and araneus diadematus. These are the two species of spiders for which the vast majority of time and money on synthetic spider silk research has been to replicate their dragline silk proteins. Yet, their draglines are not the toughest. In fact, the toughest dragline in this survey is this one right here in this white region, a non orb-web-weaving spider. This is the dragline spun by scytodes, the spitting spider. Scytodes doesn't use a web at all to catch prey. Instead, scytodes sort of lurks around and waits for prey to get close to it, and then immobilizes prey by spraying a silk-like venom onto that insect. Think of hunting with silly string. That's how scytodes forages. We don't really know why scytodes needs such a tough dragline, but it's unexpected results like this that make bio-prospecting so exciting and worthwhile. It frees us from the constraints of our imagination.
Now I'm going to mark on the toughness values for nylon fiber, bombyx -- or domesticated silkworm silk -- wool, Kevlar, and carbon fibers. And what you can see is that nearly all the spider draglines surpass them. It's the combination of strength, extensibility and toughness that makes spider silk so special, and that has attracted the attention of biomimeticists, so people that turn to nature to try to find new solutions. And the strength, extensibility and toughness of spider silks combined with the fact that silks do not elicit an immune response, have attracted a lot of interest in the use of spider silks in biomedical applications, for example, as a component of artificial tendons, for serving as guides to regrow nerves, and for scaffolds for tissue growth.
Spider silks also have a lot of potential for their anti-ballistic capabilities. Silks could be incorporated into body and equipment armor that would be more lightweight and flexible than any armor available today. In addition to these biomimetic applications of spider silks, personally, I find studying spider silks just fascinating in and of itself. I love when I'm in the laboratory, a new spider silk sequence comes in. That's just the best. (Laughter) It's like the spiders are sharing an ancient secret with me, and that's why I'm going to spend the rest of my life studying spider silk. The next time you see a spider web, please, pause and look a little closer. You'll be seeing one of the most high-performance materials known to man. To borrow from the writings of a spider named Charlotte, silk is terrific.
Thank you. (Applause)
(Applause)
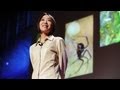
クモの壮麗さを伝え クモがもっている特徴から どれほど多くのことが学べるのかお話しします クモは本当に全世界に生息します 地球上のほとんどの場所に クモがいるのが分かります 画面上の赤い点は 北米の グレートベースン国立公園です そこで高山地帯の生命多様性を 共同研究者と研究しています これは研究場所の1か所です 青色のぼんやりとした点が見えますか? あれが研究者の1人と言えば 広大さを掴んでもらえますでしょうか こういった岩だらけの不毛地帯ですら 多くの種のクモが生息しています 岩をひっくり返すと 甲虫と組み合っている カニグモがでてきます
クモは世界中の様々な環境に分布するだけでなく 非常に多様性に富んでいます 今までに 40000種以上が 発見されています 霊長目が400種程度な一方 クモは40000種いることを グラフにするとその差を掴んでもらえるでしょうか グラフにするとその差を掴んでもらえるでしょうか 数字で2桁の違いがあるほど 霊長目よりも クモが多いのです クモは非常に古くから存在する種です この表の下には 地球誕生からの時間軸があります 目盛の数字は4百万年前から現在を 示しています 右下のゼロは 現在を表しています この表が示すように クモの起源は3億8千万年前に遡ります 全体から見ると 右下の 赤色の縦棒あたりが人類の起源です 人とチンパンジーが分化したのは たった7百万年前でしかありません
全てのクモが 生活の中で糸を生成します ほとんどのクモが生存や繁殖に 多量の糸を使います この糸は欠かすことができません とても重要なものなんです 太古のクモでさえ糸を使用しています 出糸突起のようなものを この化石のクモにも認めることができます このことから クモもクモの糸も 3億8千万年もの間 存在していることになります クモの生活のまさに全てにおいて 糸が必需品であることは 研究すればすぐに気がつきます 様々な目的に使っています 例えば 牽引糸でぶら下がる為に 繁殖時の卵を覆う為に 隠れ家を作る為に そして餌を捕まえる為に使います
糸の種類も数多くあります 例えば 画面のニワオニグモは7種類の 糸を生成できます クモの巣を見れば 多様な糸の繊維が見えてきます 巣の枠糸と放射線上の縦糸は 1つの糸種で出来ていて らせん状の捕獲部(横糸)は 2つの糸種で出来ています 線のようなフィラメントと粘球で出来ています どのようにして 一匹のクモが これほど多くの種類の糸を生成するのでしょうか? その答えを見つけるには 出糸突起部分の観察が必要です 糸は 出糸突起から吐出されます この部分を我々クモ糸の学者は 「営業窓口(先端部)」と読んでいます(笑) 学者は長時間... ちょっと 笑わないで 真剣に取り組んでいるんです(笑) ちょっと 笑わないで 真剣に取り組んでいるんです(笑) 学者は長時間昼夜を問わず クモの先端部を観察しています 画面に出ているこれを見ています 複数の繊維が出糸突起から 出ているのがわかります 各々の出糸突起に多くの糸いぼ(発射口)があるのです 各種の糸繊維が糸いぼから外に出るのですが もし繊維の根元まで辿れば 糸いぼのそれぞれが個々の糸の分泌腺に 繋がっているとわかります 分泌腺は袋のような形状をしており 中は糸タンパク質で満たされています クモの巣を作るクモを 解剖する機会がありましたら いえ 是非解剖してみてください 半透明で美しい糸分泌腺が 見れるでしょう
分泌腺の数は数百 時には数千に及ぶこともあります 分泌腺は 7種類に分類することができます その大きさ 形 そして 色までもが 異なります クモの巣を作るクモには 7種類の糸の分泌腺(絹糸腺)があり 画面に図解しました 右上1時の方向からお話しします 管状腺という絹糸腺があり 卵を包む糸のふくろを作ります 次の集合腺と鞭状腺は 混ざり クモの巣のらせん部の 粘着する糸(横線)を作ります 梨状腺は 基質に糸が着くようにする 付着剤を作ります ブドウ状腺の糸は 餌を絡めるのに使います 小瓶状腺の糸は巣に使います そして 最も研究が集中しているのが 大瓶状腺です ここの糸は枠(枠糸)と放射状の(横糸)で クモの巣を作り また ぶら下がる時に 安全にぶら下がる牽引糸となります
では糸は一体なにでできているのでしょうか? クモの糸はタンパク質のかたまりです このタンパク質のほとんどは 単一の遺伝子族でできています これは現代の多様な遺伝子が 全て単一の遺伝子族の中に組み込まれていることを意味します おそらくは クモの原種は1種類の糸しか 作っていなかったと考えられます 3億8千年の進化の過程で 糸の遺伝子が複製され分岐し 特性を持つ糸ができるプロセスが 何度も繰り返されて 現在存在する非常に多様な 種類が生成されるに至ったのです 全種の糸に共通する点が何個かあります まずはデザインですが タンパク質の長さは 一貫して 驚く程長くなっています グリシン・アラニンといったアミノ酸に富んだ とても反復的な配列を形成しています クモ糸のタンパク質がどんなものか お見せしましょう これは クロゴケグモの 牽引糸タンパク質の ごく一部です この配列を昼も夜も調べるのが たまらないんです (笑)
画面には 配列内のアミノ酸を 頭文字で示し 色を着けました グリシンを緑色のG アラニンを赤色のAで示しました ほとんどAとGが占めていることが窺えます さらに 同じ短いモチーフが何度も何度も何度も 繰り返されているのが分かりますか 例えば ポリアラニンと呼ぶ Aが繰り返されている AAAAAという箇所や GGQといったりGGYといった繰り返しがあります この繰り返す同じモチーフは 文章の中で何度も出てくる 単語であるかのようです 例えば これが1つの文とすると 緑色が多い部分や 赤色のポリアラニンの繰り返しが 何度も何度も出てきます この部分が一つ一つの糸の分子に 何百も何百も何百も 出てくるのです
同じクモが作る糸でも 配列は驚くほどに異なります 画面の上部に出ているのは コガネグモが 出した牽引糸の配列反復単位です この糸の場合 1単位の長さは短くなっています 画面の下部に出ているのは 卵を包む糸(管状糸)の タンパク質の反復配列です 全く同じクモのものですが 劇的に違うのがわかりますね ご覧の通り この遺伝子族の 多様性こそが クモ糸の美点なのです 反復単位の長さだけでなく 配列も違うことが見て取れます 配列を示すのにグリシンを緑色に アラニンを赤色にし セリンを紫色でSとしました 画面上部の反復単位は ほぼ全体が 緑と赤で構成されています 下部は 紫がほとんどを占めます 糸学者はこの反復内の配列または アミノ酸配列が糸繊維の 力学的属性と関連が無いか 調べるのです
クモが糸を使うのは完全に体外であることは 非常に都合のいいことです この特性のおかげで研究室での クモ糸の実験が簡単にできます クモが糸のタンパク質を使う 環境と全く同じ空気中で 実験ができるためです 繊維の片方を固定して引っ張る 張力テストなどの測定方法で クモ糸の属性を測定するのに 環境を作りやすいのです この図はさきほどのクモを使って 5種類の繊維で張力テストを実施し 応力歪み曲線を描いたものです 5種類の繊維がそれぞれ異なる 性質をもっているのが分かります 特に 縦軸に応力を描きました その軸にあるそれぞれの繊維の 最大応力を見てください 繊維間で大きな違いが見られます 牽引糸 あるいは 大瓶状腺からの糸が 最も高い強度を示しました 牽引糸が最強なのは枠糸と放射線状の横糸で クモの巣を作るには 糸が非常に強い必要があるからと 学者は考えています
もう1つの力 どこまで伸ばせるかという 歪みを見ましょう 最大歪みを見ると またも 繊維間で大きな違いがあります 鞭毛 または 横糸が あきらかに勝ります 鞭毛繊維は実に 当初の2倍以上の長さまで伸ばせます このように 糸繊維は応力と歪みの点で 大きく異なります らせんの状横糸にいたっては 飛来する餌の衝撃を吸収できるように 相当に柔軟でなければなりません この柔軟性を欠いた場合には 巣に虫がかかったとしても 撥ねてすぐに逃げられてしまいます もし牽引糸のみで巣を作れば 虫は跳ね返されてしまうだけでしょう しかし 非常に柔軟に伸縮する らせん状横糸があるから巣は 餌がかかった衝撃を 吸収することができるのです
クモの生成する繊維の 種類は実に多種多様です クモの道具箱と呼んでいます 環境に応じてクモは これらを使い分けるのです では クモの種同士では どの程度差があるのでしょうか? 同じ種類の糸の特性はクモの種類によって違うのでしょうか? この研究領域はまだそれほど 進んでいませんが 少しだけお見せします これは21種類のクモの 牽引糸の靭性(切れにくさ)を 比較した図です 巣を作る種と作らない種の 両方を含んでいます コガネグモのように巣を作るクモが 最も高い靱性の牽引糸を持っているという 仮説があります 飛来する餌を捉える必要があるからです この表は糸の靱性を示した図です 点が上に上がるほど 糸が切れにくくなります
ここに示した21種のクモは 系統発生の進化木に従って遺伝子的に繋がっています 右側に描かれている 巣を作る種の背景は黄色にしました 2つの赤い矢印が指しているのは アメリカジョロウグモとニワオニグモの 牽引糸の靱性(切れにくさ)を 座標に示したものです この2種のクモの作る 牽引糸のタンパク質構成を 人工合成する研究に ほとんどの 主要な資金と時間が集中しています しかし この2種類のクモの牽引糸が最強なわけではなく 実際には この調査での最強の牽引糸は 左上白背景の種で 巣を作らないクモでした これは毒吹グモのヤマシログモ科が 紡いだ牽引糸です ヤマシログモ類は餌を捕まえるのに 網を使わず そのかわりに 網に忍んで獲物に近づき 糸状の毒を虫に吹きかけて 動けなくするのです 「クモ糸射出のおもちゃ」で狩りをする感じです これがこのクモの餌の集め方です 学者はヤマシログモが なぜこんな強力な牽引糸を必要なのか分かっていません しかしこのような予期しない結果こそが 生物調査を非常に興奮させ価値あるものにします 生物を調べることで人の想像力の 限界を超えることができるのです
次に 様々な繊維の持つ 靱性(切れにくさ)で図に線を引いてみました 上から順に ナイロン カイコ(家蚕) 羊毛 ケブラー繊維 炭素繊維 の線です 殆ど全てのクモ牽引糸が 靱性で勝っていますね 応力と歪みと靱性の組み合わせこそが クモの糸を特別なものにし 生体模倣科学者の注目を浴び 多くの人が自然から新しい方法を 探ろうとするのです クモ糸が応力と歪みと靱性を 持っていながらも同時に 免疫反応の心配がないことから 生物医学への適応に 多くの注目が集まっています 例えば人工腱の一部として 神経を再建することに使い 組織が作られる土台として 使うような応用がありえます
クモの糸は防弾性材質としても 多くの可能性を秘めています 糸繊維を防弾着や 装甲具に組み込んで 現存するどんな防具より 軽く柔軟なものが作れます このような 生体模倣的な活用に加えて 個人的にはクモの糸の研究 そのものに魅力を感じ クモの糸それ自体が魅力的なのです 私には 研究室で 見たことの無いクモ糸の 配列を発見する瞬間がたまりません 最高なんです (笑) その瞬間はまるでクモが古代の秘密を 教えてくれているようです 故に 私は生涯クモの糸の 研究をするつもりです 今度クモの巣を見かけたら 立ち止まって 近づいて見てください 人類が知り得る最も強力な 材質の一つを見ることになります 『シャーロットのおくりもの』の クモのシャーロットが紡いだように クモの糸は「すばらしい」です
ありがとうございます(拍手)
(拍手)
品詞分類
- 主語
- 動詞
- 助動詞
- 準動詞
- 関係詞等
TED 日本語
TED Talks
関連動画
古代岩石が変える生命の起源説タラ・ジョキッチ
2019.10.3040億年の進化を6分で説明 | TED Talkプロサンタ・チャクラバーティ
おすすめ 12018.07.06深海の謎は生命への理解を変えるカレン・ロイド
2018.02.28進化で勝ち残り、大絶滅を生き延びる方法ローレン・サラン
2017.11.21恐竜の化石探しを通して知った、宇宙における人類ケネス・ラコバラ
2016.05.17思わず息を呑む生後21日間のミツバチの姿アナンド・ヴァルマー
2015.05.11スピノサウルスを発掘するまでニザール・イブラヒム
2015.04.24自ら治療する蝶ジャープ・デ=ローデ
2015.02.09準知的粘菌が人類に教えてくれることヘザー・バーネット
2014.07.17ホタルの愛と偽りサラ・ルイス
2014.07.01ヒトフェロモンのうさん臭い謎トリストラム・ワイアット
2014.05.15脳や癌細胞とインターネット ― アリ達が教えてくれる事デボラ・ゴードン
2014.05.13自殺するコオロギ、ゾンビ化するゴキブリ、その他の寄生生物にまつわる話エド・ヨン
おすすめ 32014.03.26ハチが消えつつある理由マーラ・スピヴァク
TED人気動画2013.09.17絶滅種 カモノハシガエル、タスマニアン・タイガーの再生計画マイケル・アーチャー
2013.06.27ハエはどうやって飛ぶの?マイケル・ディキンソン
2013.02.22
洋楽 おすすめ
RECOMMENDS
洋楽歌詞
ダイナマイトビーティーエス
洋楽最新ヒット2020.08.20ディス・イズ・ミーグレイテスト・ショーマン・キャスト
洋楽人気動画2018.01.11グッド・ライフGイージー、ケラーニ
洋楽人気動画2017.01.27ホワット・ドゥ・ユー・ミーン?ジャスティン・ビーバー
洋楽人気動画2015.08.28ファイト・ソングレイチェル・プラッテン
洋楽人気動画2015.05.19ラヴ・ミー・ライク・ユー・ドゥエリー・ゴールディング
洋楽人気動画2015.01.22アップタウン・ファンクブルーノ・マーズ、マーク・ロンソン
洋楽人気動画2014.11.20ブレイク・フリーアリアナ・グランデ
洋楽人気動画2014.08.12ハッピーファレル・ウィリアムス
ポップス2014.01.08カウンティング・スターズワンリパブリック
ロック2013.05.31ア・サウザンド・イヤーズクリスティーナ・ペリー
洋楽人気動画2011.10.26ユー・レイズ・ミー・アップケルティック・ウーマン
洋楽人気動画2008.05.30ルーズ・ユアセルフエミネム
洋楽人気動画2008.02.21ドント・ノー・ホワイノラ・ジョーンズ
洋楽人気動画2008.02.15オンリー・タイムエンヤ
洋楽人気動画2007.10.03ミス・ア・シングエアロスミス
ロック2007.08.18タイム・トゥ・セイ・グッバイサラ・ブライトマン
洋楽人気動画2007.06.08シェイプ・オブ・マイ・ハートスティング
洋楽人気動画2007.03.18ウィ・アー・ザ・ワールド(U.S.A. フォー・アフリカ)マイケル・ジャクソン
洋楽人気動画2006.05.14ホテル・カリフォルニアイーグルス
ロック2005.07.06