
TED日本語
TED Talks(英語 日本語字幕付き動画)
TED日本語 - マイケル・ディキンソン: ハエはどうやって飛ぶの?
TED Talks
ハエはどうやって飛ぶの?
How a fly flies
マイケル・ディキンソン
Michael Dickinson
内容
昆虫が飛べるようになったことは、生物の進化において最も重要な出来事の一つに違いない。マイケル・ディキンソンが、身近な昆虫、ハエの飛行について語ります。小さな羽・巧みな羽ばたき・大きな力筋が生み出す、すばしこくて力強いハエの飛行。でも、一番の秘密はその脳の中に隠されています。(撮影地: TEDxCaltech)
字幕
SCRIPT
Script
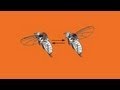
I grew up watching Star Trek. I love Star Trek. Star Trek made me want to see alien creatures, creatures from a far-distant world. But basically, I figured out that I could find those alien creatures right on Earth.
And what I do is I study insects. I'm obsessed with insects, particularly insect flight. I think the evolution of insect flight is perhaps one of the most important events in the history of life. Without insects, there'd be no flowering plants. Without flowering plants, there would be no clever, fruit-eating primates giving TED Talks.
(Laughter)
Now, David and Hidehiko and Ketaki gave a very compelling story about the similarities between fruit flies and humans, and there are many similarities, and so you might think that if humans are similar to fruit flies, the favorite behavior of a fruit fly might be this, for example -- (Laughter) but in my talk, I don't want to emphasize on the similarities between humans and fruit flies, but rather the differences, and focus on the behaviors that I think fruit flies excel at doing.
And so I want to show you a high-speed video sequence of a fly shot at 7,000 frames per second in infrared lighting, and to the right, off-screen, is an electronic looming predator that is going to go at the fly. The fly is going to sense this predator. It is going to extend its legs out. It's going to sashay away to live to fly another day. Now I have carefully cropped this sequence to be exactly the duration of a human eye blink, so in the time that it would take you to blink your eye, the fly has seen this looming predator, estimated its position, initiated a motor pattern to fly it away, beating its wings at 220 times a second as it does so. I think this is a fascinating behavior that shows how fast the fly's brain can process information.
Now, flight -- what does it take to fly? Well, in order to fly, just as in a human aircraft, you need wings that can generate sufficient aerodynamic forces, you need an engine sufficient to generate the power required for flight, and you need a controller, and in the first human aircraft, the controller was basically the brain of Orville and Wilbur sitting in the cockpit.
Now, how does this compare to a fly? Well, I spent a lot of my early career trying to figure out how insect wings generate enough force to keep the flies in the air. And you might have heard how engineers proved that bumblebees couldn't fly. Well, the problem was in thinking that the insect wings function in the way that aircraft wings work. But they don't. And we tackle this problem by building giant, dynamically scaled model robot insects that would flap in giant pools of mineral oil where we could study the aerodynamic forces. And it turns out that the insects flap their wings in a very clever way, at a very high angle of attack that creates a structure at the leading edge of the wing, a little tornado-like structure called a leading edge vortex, and it's that vortex that actually enables the wings to make enough force for the animal to stay in the air. But the thing that's actually most -- so, what's fascinating is not so much that the wing has some interesting morphology. What's clever is the way the fly flaps it, which of course ultimately is controlled by the nervous system, and this is what enables flies to perform these remarkable aerial maneuvers.
Now, what about the engine? The engine of the fly is absolutely fascinating. They have two types of flight muscle: so-called power muscle, which is stretch-activated, which means that it activates itself and does not need to be controlled on a contraction-by-contraction basis by the nervous system. It's specialized to generate the enormous power required for flight, and it fills the middle portion of the fly, so when a fly hits your windshield, it's basically the power muscle that you're looking at. But attached to the base of the wing is a set of little, tiny control muscles that are not very powerful at all, but they're very fast, and they're able to reconfigure the hinge of the wing on a stroke-by-stroke basis, and this is what enables the fly to change its wing and generate the changes in aerodynamic forces which change its flight trajectory. And of course, the role of the nervous system is to control all this.
So let's look at the controller. Now flies excel in the sorts of sensors that they carry to this problem. They have antennae that sense odors and detect wind detection. They have a sophisticated eye which is the fastest visual system on the planet. They have another set of eyes on the top of their head. We have no idea what they do. They have sensors on their wing. Their wing is covered with sensors, including sensors that sense deformation of the wing. They can even taste with their wings. One of the most sophisticated sensors a fly has is a structure called the halteres. The halteres are actually gyroscopes. These devices beat back and forth about 200 hertz during flight, and the animal can use them to sense its body rotation and initiate very, very fast corrective maneuvers. But all of this sensory information has to be processed by a brain, and yes, indeed, flies have a brain, a brain of about 100,000 neurons.
Now several people at this conference have already suggested that fruit flies could serve neuroscience because they're a simple model of brain function. And the basic punchline of my talk is, I'd like to turn that over on its head. I don't think they're a simple model of anything. And I think that flies are a great model. They're a great model for flies. (Laughter)
And let's explore this notion of simplicity. So I think, unfortunately, a lot of neuroscientists, we're all somewhat narcissistic. When we think of brain, we of course imagine our own brain. But remember that this kind of brain, which is much, much smaller? instead of 100 billion neurons, it has 100,000 neurons- but this is the most common form of brain on the planet and has been for 400 million years. And is it fair to say that it's simple? Well, it's simple in the sense that it has fewer neurons, but is that a fair metric? And I would propose it's not a fair metric. So let's sort of think about this. I think we have to compare -- (Laughter) - we have to compare the size of the brain with what the brain can do. So I propose we have a Trump number, and the Trump number is the ratio of this man's behavioral repertoire to the number of neurons in his brain. We'll calculate the Trump number for the fruit fly. Now, how many people here think the Trump number is higher for the fruit fly?
(Applause)
It's a very smart, smart audience. Yes, the inequality goes in this direction, or I would posit it.
Now I realize that it is a little bit absurd to compare the behavioral repertoire of a human to a fly. But let's take another animal just as an example. Here's a mouse. A mouse has about 1,000 times as many neurons as a fly. I used to study mice. When I studied mice, I used to talk really slowly. And then something happened when I started to work on flies. (Laughter) And I think if you compare the natural history of flies and mice, it's really comparable. They have to forage for food. They have to engage in courtship. They have sex. They hide from predators. They do a lot of the similar things. But I would argue that flies do more. So for example, I'm going to show you a sequence, and I have to say, some of my funding comes from the military, so I'm showing this classified sequence and you can not discuss it outside of this room. Okay? So I want you to look at the payload at the tail of the fruit fly. Watch it very closely, and you'll see why my six-year-old son now wants to be a neuroscientist. Wait for it. Pshhew. So at least you'll admit that if fruit flies are not as clever as mice, they're at least as clever as pigeons. (Laughter)
Now, I want to get across that it's not just a matter of numbers but also the challenge for a fly to compute everything its brain has to compute with such tiny neurons. So this is a beautiful image of a visual interneuron from a mouse that came from Jeff Lichtman's lab, and you can see the wonderful images of brains that he showed in his talk. But up in the corner, in the right corner, you'll see, at the same scale, a visual interneuron from a fly. And I'll expand this up. And it's a beautifully complex neuron. It's just very, very tiny, and there's lots of biophysical challenges with trying to compute information with tiny, tiny neurons.
How small can neurons get? Well, look at this interesting insect. It looks sort of like a fly. It has wings, it has eyes, it has antennae, its legs, complicated life history, it's a parasite, it has to fly around and find caterpillars to parasatize, but not only is its brain the size of a salt grain, which is comparable for a fruit fly, it is the size of a salt grain. So here's some other organisms at the similar scale. This animal is the size of a paramecium and an amoeba, and it has a brain of 7,000 neurons that's so small -- you know these things called cell bodies you've been hearing about, where the nucleus of the neuron is? This animal gets rid of them because they take up too much space. So this is a session on frontiers in neuroscience. I would posit that one frontier in neuroscience is to figure out how the brain of that thing works.
But let's think about this. How can you make a small number of neurons do a lot? And I think, from an engineering perspective, you think of multiplexing. You can take a hardware and have that hardware do different things at different times, or have different parts of the hardware doing different things. And these are the two concepts I'd like to explore. And they're not concepts that I've come up with, but concepts that have been proposed by others in the past.
And one idea comes from lessons from chewing crabs. And I don't mean chewing the crabs. I grew up in Baltimore, and I chew crabs very, very well. But I'm talking about the crabs actually doing the chewing. Crab chewing is actually really fascinating. Crabs have this complicated structure under their carapace called the gastric mill that grinds their food in a variety of different ways. And here's an endoscopic movie of this structure. The amazing thing about this is that it's controlled by a really tiny set of neurons, about two dozen neurons that can produce a vast variety of different motor patterns, and the reason it can do this is that this little tiny ganglion in the crab is actually inundated by many, many neuromodulators. You heard about neuromodulators earlier. There are more neuromodulators that alter, that innervate this structure than actually neurons in the structure, and they're able to generate a complicated set of patterns. And this is the work by Eve Marder and her many colleagues who've been studying this fascinating system that show how a smaller cluster of neurons can do many, many, many things because of neuromodulation that can take place on a moment-by-moment basis. So this is basically multiplexing in time. Imagine a network of neurons with one neuromodulator. You select one set of cells to perform one sort of behavior, another neuromodulator, another set of cells, a different pattern, and you can imagine you could extrapolate to a very, very complicated system.
Is there any evidence that flies do this? Well, for many years in my laboratory and other laboratories around the world, we've been studying fly behaviors in little flight simulators. You can tether a fly to a little stick. You can measure the aerodynamic forces it's creating. You can let the fly play a little video game by letting it fly around in a visual display. So let me show you a little tiny sequence of this. Here's a fly and a large infrared view of the fly in the flight simulator, and this is a game the flies love to play. You allow them to steer towards the little stripe, and they'll just steer towards that stripe forever. It's part of their visual guidance system. But very, very recently, it's been possible to modify these sorts of behavioral arenas for physiologies. So this is the preparation that one of my former post-docs, Gaby Maimon, who's now at Rockefeller, developed, and it's basically a flight simulator but under conditions where you actually can stick an electrode in the brain of the fly and record from a genetically identified neuron in the fly's brain. And this is what one of these experiments looks like. It was a sequence taken from another post-doc in the lab, Bettina Schnell. The green trace at the bottom is the membrane potential of a neuron in the fly's brain, and you'll see the fly start to fly, and the fly is actually controlling the rotation of that visual pattern itself by its own wing motion, and you can see this visual interneuron respond to the pattern of wing motion as the fly flies. So for the first time we've actually been able to record from neurons in the fly's brain while the fly is performing sophisticated behaviors such as flight. And one of the lessons we've been learning is that the physiology of cells that we've been studying for many years in quiescent flies is not the same as the physiology of those cells when the flies actually engage in active behaviors like flying and walking and so forth. And why is the physiology different? Well it turns out it's these neuromodulators, just like the neuromodulators in that little tiny ganglion in the crabs. So here's a picture of the octopamine system. Octopamine is a neuromodulator that seems to play an important role in flight and other behaviors. But this is just one of many neuromodulators that's in the fly's brain. So I really think that, as we learn more, it's going to turn out that the whole fly brain is just like a large version of this stomatogastric ganglion, and that's one of the reasons why it can do so much with so few neurons.
Now, another idea, another way of multiplexing is multiplexing in space, having different parts of a neuron do different things at the same time. So here's two sort of canonical neurons from a vertebrate and an invertebrate, a human pyramidal neuron from Ramon y Cajal, and another cell to the right, a non-spiking interneuron, and this is the work of Alan Watson and Malcolm Burrows many years ago, and Malcolm Burrows came up with a pretty interesting idea based on the fact that this neuron from a locust does not fire action potentials. It's a non-spiking cell. So a typical cell, like the neurons in our brain, has a region called the dendrites that receives input, and that input sums together and will produce action potentials that run down the axon and then activate all the output regions of the neuron. But non-spiking neurons are actually quite complicated because they can have input synapses and output synapses all interdigitated, and there's no single action potential that drives all the outputs at the same time. So there's a possibility that you have computational compartments that allow the different parts of the neuron to do different things at the same time.
So these basic concepts of multitasking in time and multitasking in space, I think these are things that are true in our brains as well, but I think the insects are the true masters of this. So I hope you think of insects a little bit differently next time, and as I say up here, please think before you swat.
(Applause)
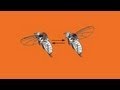
僕はスタートレックを見て育った。スタートレックが好きでたまらない。 好きが高じて、エイリアンに会いたいと思うようになった 遠い世界から来た生物にね まあ 自分が出会えるのはせいぜい 地球上の変な生物くらいだろうと分かってたけどね
大人になって 僕は昆虫の研究者になった 僕は昆虫に夢中で、特にその飛び方に興味を持ってる 昆虫の飛行という進化は、おそらく 生命の歴史において最も重要な出来事のひとつだろう 昆虫がいなければ 被子植物も出現せず 被子植物が存在しなければ 賢いサルたちが TED で話をすることもなかっただろうからね
(笑い声)
さて 先ほどの デヴィッドとヒデヒコとケタキの3人が とても興味深い話をした ミバエと人間の類似点について 確かに、類似点がたくさんあるから ミバエと人間が似ているのかと思う人がいるかもしれない たとえば ミバエの好きな行動はこれだとか -- (笑い声) でも、今日は、人間とミバエの類似点ではなく 両者の違いについて、 そして、ミバエの長所について紹介しようと思う
まずは、高速カメラで撮影したものをお見せしましょう 毎秒7000コマの赤外線映像だ 画面から外れた右の方に捕食者が迫っていて ハエを狙っている ハエが捕食者を察知している 脚を伸ばして スッと逃げる 命拾いしたね この映像は人間の瞬きと 同じ時間にカットしてある 人間が一回瞬きをする間に ミバエが捕食者を発見して 位置を確認して飛び去るための筋肉運動を開始した 毎秒220回も羽ばたきながら これはハエの脳がいかに高速に 情報を処理しているかを示す興味深い動きだ
では飛行だけど -- 飛ぶには何が必要かな? 飛ぶためには飛行機と同じように 十分な空気力を生み出す翼が必要だ 飛行するための力を生み出す十分なエンジンと 制御装置も必要だ 人類初の飛行機の制御装置は、基本的に コクピットのライト兄弟の脳みそだった
これをハエと比べるとどうだろう 研究者になりたての頃 僕は ハエの羽がどのようにして十分な浮力を生み出しているか、との謎を解き明かそうと必死で研究していた 聞いたことないかな?航空力学の計算によると マルハナバチは飛べないことになるって 昆虫の羽の働きを飛行機と同じように 考えていたのが間違いだったんだ この問題を解くため 動力学的に拡大した 巨大な昆虫のロボットを作って 実験を行った ロボットを大きな鉱物油の中で羽ばたかせ 空気力学の力を観察した そこで分かったんだけど 昆虫の羽ばたき方は とても賢くて 大きな迎角によって羽の先端に 前縁渦と呼ばれる竜巻状の流れを生み出す この渦によって昆虫の羽は 十分な浮力を生み出すことができるんだ しかしながら、一番興味深いのは 羽の形ではなくて羽の形状組織だ。 なんて賢い羽の動かし方をしているんだろう! 羽の動きは、結局のところ、神経で制御されていて そのおかげで ハエは自在に飛び回れる 注目すべきは、飛行の巧みさ
じゃあ エンジンはどうだろう? ハエのエンジンはとても面白い ハエには2種類の飛行筋があるんだ 力筋と呼ばれるものは 伸展活性型なんだけど それ自体が活性型なので、 伸縮の度に神経による制御を必要としない 飛ぶための大きな力を生み出す目的に特化した筋肉で ハエの胸部を占めている それ故、フロントガラスにハエが衝突した時 見えるのは ほとんどこの力筋なんだ でもその他に 羽の付け根には 力は弱いけど 反応が非常に速い小さな制御筋があって 羽ばたきごとに 羽の蝶番を 調整することができる これによって ハエは羽ばたきを調整して 空気を操って 違う方向に飛ぶことができる これを全て制御しているのが 神経系統だ
では制御装置を見てみよう ハエは優れたセンサーの持ち主だが それがいいことばかりとは限らない 彼らは、匂いと風向きを感知するアンテナの持ち主だ 彼らは高度な眼を持っている それは、地上最速の視覚システムなんだ さらに 頭頂部にも一対の眼がある その眼はどんな役割りをはたすのか、私達がさっぱり分からない。 それらが、羽にセンサーがある。 羽がセンサーで覆われていて、 そのセンサーの中で、形状の変形を感知するセンサーもある。 羽で味覚まで備えている ハエのセンサーの中でも優れているのが 平均棍と呼ばれる器官だ 平均棍はジャイロスコープのような器官で 飛行中は200ヘルツで振り子運動する ハエはこれを使って 体の回転を感知し 素早く 飛行姿勢を修正することができる しかし センサーからの情報は、すべて脳で処理しなければならない そうだよ ハエにも脳があるんだ 10万個の神経細胞からなる脳がね
このコンファレンスの出席者の中にも ミバエの脳機能は単純だから 神経科学の研究に適しているなんて言う人がいるようだね でも、侮るなかれ! 僕に言わせれば、それは全くの勘違いだよ ハエの脳は単純なんかじゃないと思うんだ 素晴らしいモデルだ ハエにしてみればね (笑い声)
では なぜ単純だと思われてしまうのだろうか? 残念ながら、神経科学者はね、 僕たちは自己中に考えがちだと思う 「脳」というと 自分たちの脳を基準に考える でも、思い出してみて。こんな脳だよ。 こんなとても小さい脳が -- 1000億個ではなく 10万個の細胞しかない -- 地球上で最も一般的な形態であって それは4億年前から続いている これを単純だと言い切って良いのかな? 神経細胞の数は確かに少ない でもその基準で大丈夫なのか? 僕は違うと思う ちょっとこれについて考えてみようまずこの比較から見てもらおう -- (笑い声) 脳の大きさとでその脳が何ができるか 比較しなくちゃならない トランプ数という指数があるとしよう トランプ数の行動パターンの数を 神経細胞の数で割った値だ 同様にハエについても計算してみる どうだろう! ハエのトランプ数の方が高い と思う人いますか?
(拍手)
今日お越しの皆さんは頭がいいね そうなんだ、大きさと機能は必ずしも比例しない
ハエと人間の行動パターンの数を比べるのは あまり合理的じゃないかも知れない じゃあ 他の動物はどうだろう?たとえばネズミ ネズミはハエの1000倍の神経細胞をもっている 昔 ネズミを研究していた。その当時は 僕だって ゆっくり喋っていた でもハエの研究を始めてから 何かが変わったんだ (笑い声) ハエとネズミは 博物学的に見て共通点がある 食べ物を漁ったり 求愛行動をしたり 交尾をしたり 捕食者から隠れたりする 共通点はたくさんあるけど ハエの方が行動パターンが多いと 僕は思う たとえば これから見せる映像 ああ、ちょっと言っておかなくちゃ。実を言うと、僕は、軍から一部、資金援助を受けているんだ。 だから、この極秘映像を見たことは内緒だよ 決して誰にも口外しないでね! OK? ハエのお尻にぶら下がっている 爆弾に注意しながら見てほしい 近くに来て、よく見てごらん。 うちの6歳の息子が 神経科学者になると 言い出した理由が分かるから タイミングを計って ドーン 少なくとも、ハエがネズミほど賢くない、という事はお分かりでしょう。 少なくとも、ハト並の知能を持っている。(笑い声)
まずはね、それは、神経細胞の数だけじゃなく、 ハエはあんなに小さな神経細胞で 全ての情報を処理しているんだ、という事をお伝えしたい。 これはジェフ・リックマンから借りてきた ネズミの神経細胞の美しい写真だ その脳の美しい画像を見られるよ 彼は、講演で画像を見せたんだ。 右端の上にあるのは 同じ縮尺で示された ハエの神経細胞だ 拡大してみよう 素晴らしく細かな神経細胞だよね。 生物物理学的には こんな小さな神経細胞で 大量の情報を処理しようとするんだ。
神経細胞はどこまで小さくなれるのか?ここに面白い虫がいる 見た目はハエに似ているね 羽・眼・アンテナ・脚がありライフサイクルも複雑 実は毛虫に寄生する寄生虫なんだ そして、それは、塩粒と同じ大きさの脳というだけでなく、 ミバエと比べると 体全体が塩粒くらいの大きさしかない 他の生命体と大きさを比べてみよう ゾウリムシやアメーバと同じくらいの大きさだけど 非常に小さな7000個の神経細胞からなる脳を持っている 君達、聞いた事があるかな?これらの細胞体と呼ばれるもので 神経細胞の核は細胞体に入っているんだ。 これは、この大きさでさえ、あまりに場所を取りすぎるから取り除かれている 今回のテーマは神経科学の最前線なんだ ある神経科学の最前線では、脳が機能する仕組みを解き明かすことができる、と仮定してみよう含まれているだろう
一緒に考えみよう。少数の神経細胞でたくさんの処理を行うにはどうすればいいのか? 工学的観点から見ると 君たちは、多重化と考えるかもしれないね。 ある機器を用いて、その装置に 違う時間にに違う処理をさせる 又は、その装置の他の部分で違う処理をさせるんだ。 僕が調べてみたいのは2つある これらの概念は僕が思いついたものではなく 他の人たちが過去に提唱していたものだ
その一つは カニの噛み方からヒントを得ている 僕らがカニを噛むんじゃないよ 僕はボルチモア育ちだからカニを噛むのは超得意だけどね いま話してるのはカニ自身の咀嚼行動についてだ カニの咀嚼行動は実に興味深い カニの甲羅の下には複雑な器官があって 咀嚼器と呼ばれる いろんな風に食べ物をかみ砕くことができる これは咀嚼器の内視鏡映像 咀嚼器の何がすごいかというと わずか20個程度の神経細胞で 多様な筋肉運動のパターンを作り出している点だ これが可能なのは カニの小さな神経節が 多種の神経調節物質に浸されているからだ 神経調整物質については、さっきのスピーチにも登場したね 神経細胞よりも多くの 神経調節物質があって それを変化させたり、実際に、その器官の神経細胞の数よりも多くを刺激したりしている そして、その事が 複雑な運動パターンを生み出す事を可能にしている ここにイブ・マーダーと仲間たちの長年の研究成果がある 彼らは素晴らしい組織の研究していて それは、どんな少数の神経細胞の集まりでも いろんなことができる という事を明らかにしているんだ というのも、根本的に、神経調節は刻々と発生するからね すなわち、これは基本的には、時間的多重化という事だ 1つの神経調節物質に、1つの神経細胞網があると想像してごらん。 ある神経調整物質といくつかの細胞にある行動を割り当てて 別の神経調節物質と細胞に別の行動を割り当てていくと 非常に複雑な運動パターンが可能になることが これで想像できるだろう
ハエも同じだろうか? 長年に渡り 私の研究所でも、世界中の研究所でも 小さな飛行シミュレーターを使ってハエの行動を研究してきた ハエを棒の先にくっつけて 空気力を計測するんだ ハエがテレビゲームをやっているような格好になる 映像の中にハエを飛ばしておくことによって この続きを少しお見せしましょう 一匹のハエがいるね 飛行シミュレーターの中のハエの大きな赤外線映像で、 これは、ハエが好むゲームの一つなんだ。 ハエが 小さな縞模様の方に飛んでいくように仕向けると ハエは 長時間 これを続けようとする。 ハエの視線誘導システムにもともと組み込まれている働きなんだ でも、ごく最近では こうした行動領域を 生理学的に変更することが可能になっている これは、以前、ここでギャビー・メイモンがポスドクだった頃に研究していた準備段階のものなんだけど、 今、彼はロックフェラー大学でこれを完成させたんだ。 それは 単なる飛行シミュレーターではなく ハエの脳に電極を挿入して 遺伝子的に特定された神経細胞の 電流を記録することができる これらの実験の様子のひとつがこちら。 実験室では、ポスドクから後任のポスドクへ実験が引き継がれていたんだ。 後任者は ベッティナ・シュネル。 画面下の緑の線は ハエの脳細胞の膜電位だ。 ほら、ハエが飛び始めたよ。 模様の回転は ハエ自身が羽の動きで制御している 視覚介在神経が見えるよね ハエが飛ぶ時に、 これが羽の運動パターンに反応するんだ だから、初めて我々が ハエの脳神経の電流を記録することができるようになったんだ 飛行など という高度な行動を行っているときでもね その結果、我々が学んだ事の一つは 細胞生理学だ。 我々は長年に渡って、 静止状態のハエの神経細胞を研究してきたんだけど、 そこには 生理学的な違いがある 彼らが運動中の時のハエの神経細胞では- 飛行や歩行などの運動って事なんだけど。 なぜ生理学的な違いが生じるのか? 実はね、神経調節物質が重要な役割を果たしていることが分かったんだ。 カニの場合と同様 ここでも これはオクトパミン系の写真だ オクトパミンは神経調節物質だ。 それは、飛行などの行動に重要な役割を果たすらしい これは、多くの神経調節物質の一つだ そう、ハエの脳だよ だから研究を進めていけば その事が明らかになるだろう。 ハエの脳全体が、カニの咀嚼器の神経節と同じようであり、 そして、どうして少ない神経細胞で多くの処理を行えるのか、という理由の一つ
もう一つの考え方、そして他の多重化の方法について考えてみよう 空間的多重化では 神経細胞の別々の部分で 同時に別々の処理を行う これは2種類の標準的な神経細胞 脊椎動物と無脊椎動物からの ラモン・イ・カハールによる人間の錐体細胞の図 右にある他の細胞は、ノンスパイキング介在神経細胞の図 そして、これは、ずっと以前のアラン・ワトソンとマルコム・バロウズの業績なんだ マルコムは面白い考え方を提案した ある昆虫の神経細胞に基づいていて、 活動電位を発火させないんだ。 それがノンスパイキング細胞だ。 僕たちの脳神経細胞の様な 、一般的な神経細胞は 樹状突起と呼ばれる部分があって そこで受けた入力がたまると 活動電位が発生して 軸索突起を伝わり 神経細胞の出力部分を活性化させる でも、ノンスパイキング神経細胞はもっと複雑だ というのも、入力シナプスと出力シナプスが複雑に絡み合っていて 全ての出力を駆動する単一の活動電位というものがない つまり同じ時間に全てを出力するようにできているからなんだね。 とすれば ある可能性が出てくる。それは計算用のブロックが区切られていて 一つの神経細胞の別々の部分で 別々の処理を行っている可能性があるという事なんだ。
時間的な多重化という これらの基本的考え、 そして 空間的な多重化は 基礎概念としては 人間の脳にも当てはまる でも 昆虫の多重化の方が発達しているんだ 今日の話で 昆虫に対する考え方が変わったかな? 今度ハエたたきを振り回す前に今日の話を思い出してください
(拍手)
品詞分類
- 主語
- 動詞
- 助動詞
- 準動詞
- 関係詞等
TED 日本語
TED Talks
関連動画
古代岩石が変える生命の起源説タラ・ジョキッチ
2019.10.3040億年の進化を6分で説明 | TED Talkプロサンタ・チャクラバーティ
おすすめ 12018.07.06深海の謎は生命への理解を変えるカレン・ロイド
2018.02.28進化で勝ち残り、大絶滅を生き延びる方法ローレン・サラン
2017.11.21恐竜の化石探しを通して知った、宇宙における人類ケネス・ラコバラ
2016.05.17思わず息を呑む生後21日間のミツバチの姿アナンド・ヴァルマー
2015.05.11スピノサウルスを発掘するまでニザール・イブラヒム
2015.04.24自ら治療する蝶ジャープ・デ=ローデ
2015.02.09準知的粘菌が人類に教えてくれることヘザー・バーネット
2014.07.17ホタルの愛と偽りサラ・ルイス
2014.07.01ヒトフェロモンのうさん臭い謎トリストラム・ワイアット
2014.05.15脳や癌細胞とインターネット ― アリ達が教えてくれる事デボラ・ゴードン
2014.05.13自殺するコオロギ、ゾンビ化するゴキブリ、その他の寄生生物にまつわる話エド・ヨン
おすすめ 32014.03.26ハチが消えつつある理由マーラ・スピヴァク
TED人気動画2013.09.17絶滅種 カモノハシガエル、タスマニアン・タイガーの再生計画マイケル・アーチャー
2013.06.27フンコロガシの踊りマーカス・バーン
2012.12.13
洋楽 おすすめ
RECOMMENDS
洋楽歌詞
ダイナマイトビーティーエス
洋楽最新ヒット2020.08.20ディス・イズ・ミーグレイテスト・ショーマン・キャスト
洋楽人気動画2018.01.11グッド・ライフGイージー、ケラーニ
洋楽人気動画2017.01.27ホワット・ドゥ・ユー・ミーン?ジャスティン・ビーバー
洋楽人気動画2015.08.28ファイト・ソングレイチェル・プラッテン
洋楽人気動画2015.05.19ラヴ・ミー・ライク・ユー・ドゥエリー・ゴールディング
洋楽人気動画2015.01.22アップタウン・ファンクブルーノ・マーズ、マーク・ロンソン
洋楽人気動画2014.11.20ブレイク・フリーアリアナ・グランデ
洋楽人気動画2014.08.12ハッピーファレル・ウィリアムス
ポップス2014.01.08カウンティング・スターズワンリパブリック
ロック2013.05.31ア・サウザンド・イヤーズクリスティーナ・ペリー
洋楽人気動画2011.10.26ユー・レイズ・ミー・アップケルティック・ウーマン
洋楽人気動画2008.05.30ルーズ・ユアセルフエミネム
洋楽人気動画2008.02.21ドント・ノー・ホワイノラ・ジョーンズ
洋楽人気動画2008.02.15オンリー・タイムエンヤ
洋楽人気動画2007.10.03ミス・ア・シングエアロスミス
ロック2007.08.18タイム・トゥ・セイ・グッバイサラ・ブライトマン
洋楽人気動画2007.06.08シェイプ・オブ・マイ・ハートスティング
洋楽人気動画2007.03.18ウィ・アー・ザ・ワールド(U.S.A. フォー・アフリカ)マイケル・ジャクソン
洋楽人気動画2006.05.14ホテル・カリフォルニアイーグルス
ロック2005.07.06