
TED日本語
TED Talks(英語 日本語字幕付き動画)
TED日本語 - ナオミ・オレスケス: 科学者を信頼すべき理由
TED Talks
科学者を信頼すべき理由
Why we should trust scientists
ナオミ・オレスケス
Naomi Oreskes
内容
世界の重大な問題の多くは科学者の見解を必要としますが、なぜ私たちは科学者の言うことを信じるべきなのでしょうか。科学史の研究者であるナオミ・オレスケスは、私たちと信じることとの関係を深く考察し、科学研究に対する姿勢にまつわる3つの問題点を導き出します。さらに、私たちが科学を信頼すべき理由として、独自の根拠を示してくれます。
字幕
SCRIPT
Script
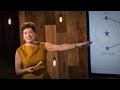
Every day we face issues like climate change or the safety of vaccines where we have to answer questions whose answers rely heavily on scientific information. Scientists tell us that the world is warming. Scientists tell us that vaccines are safe. But how do we know if they are right? Why should be believe the science? The fact is, many of us actually don't believe the science. Public opinion polls consistently show that significant proportions of the American people don't believe the climate is warming due to human activities, don't think that there is evolution by natural selection, and aren't persuaded by the safety of vaccines.
So why should we believe the science? Well, scientists don't like talking about science as a matter of belief. In fact, they would contrast science with faith, and they would say belief is the domain of faith. And faith is a separate thing apart and distinct from science. Indeed they would say religion is based on faith or maybe the calculus of Pascal's wager. Blaise Pascal was a 17th-century mathematician who tried to bring scientific reasoning to the question of whether or not he should believe in God, and his wager went like this: Well, if God doesn't exist but I decide to believe in him nothing much is really lost. Maybe a few hours on Sunday. (Laughter) But if he does exist and I don't believe in him, then I'm in deep trouble. And so Pascal said, we'd better believe in God. Or as one of my college professors said, "He clutched for the handrail of faith." He made that leap of faith leaving science and rationalism behind.
Now the fact is though, for most of us, most scientific claims are a leap of faith. We can't really judge scientific claims for ourselves in most cases. And indeed this is actually true for most scientists as well outside of their own specialties. So if you think about it, a geologist can't tell you whether a vaccine is safe. Most chemists are not experts in evolutionary theory. A physicist can not tell you, despite the claims of some of them, whether or not tobacco causes cancer. So, if even scientists themselves have to make a leap of faith outside their own fields, then why do they accept the claims of other scientists? Why do they believe each other's claims? And should we believe those claims?
So what I'd like to argue is yes, we should, but not for the reason that most of us think. Most of us were taught in school that the reason we should believe in science is because of the scientific method. We were taught that scientists follow a method and that this method guarantees the truth of their claims. The method that most of us were taught in school, we can call it the textbook method, is the hypothetical deductive method. According to the standard model, the textbook model, scientists develop hypotheses, they deduce the consequences of those hypotheses, and then they go out into the world and they say, "Okay, well are those consequences true?" Can we observe them taking place in the natural world? And if they are true, then the scientists say, "Great, we know the hypothesis is correct."
So there are many famous examples in the history of science of scientists doing exactly this. One of the most famous examples comes from the work of Albert Einstein. When Einstein developed the theory of general relativity,one of the consequences of his theory was that space-time wasn't just an empty void but that it actually had a fabric. And that that fabric was bent in the presence of massive objects like the sun. So if this theory were true then it meant that light as it passed the sun should actually be bent around it. That was a pretty startling prediction and it took a few years before scientists were able to test it but they did test it in 1919, and lo and behold it turned out to be true. Starlight actually does bend as it travels around the sun. This was a huge confirmation of the theory. It was considered proof of the truth of this radical new idea, and it was written up in many newspapers around the globe.
Now, sometimes this theory or this model is referred to as the deductive-nomological model, mainly because academics like to make things complicated. But also because in the ideal case, it's about laws. So nomological means having to do with laws. And in the ideal case, the hypothesis isn't just an idea: ideally, it is a law of nature. Why does it matter that it is a law of nature? Because if it is a law, it can't be broken. If it's a law then it will always be true in all times and all places no matter what the circumstances are. And all of you know of at least one example of a famous law: Einstein's famous equation, E=MC2, which tells us what the relationship is between energy and mass. And that relationship is true no matter what.
Now, it turns out, though, that there are several problems with this model. The main problem is that it's wrong. It's just not true. (Laughter) And I'm going to talk about three reasons why it's wrong. So the first reason is a logical reason. It's the problem of the fallacy of affirming the consequent. So that's another fancy, academic way of saying that false theories can make true predictions. So just because the prediction comes true doesn't actually logically prove that the theory is correct. And I have a good example of that too, again from the history of science. This is a picture of the Ptolemaic universe with the Earth at the center of the universe and the sun and the planets going around it. The Ptolemaic model was believed by many very smart people for many centuries. Well, why? Well the answer is because it made lots of predictions that came true. The Ptolemaic system enabled astronomers to make accurate predictions of the motions of the planet, in fact more accurate predictions at first than the Copernican theory which we now would say is true. So that's one problem with the textbook model. A second problem is a practical problem, and it's the problem of auxiliary hypotheses. Auxiliary hypotheses are assumptions that scientists are making that they may or may not even be aware that they're making. So an important example of this comes from the Copernican model, which ultimately replaced the Ptolemaic system. So when Nicolaus Copernicus said, actually the Earth is not the center of the universe, the sun is the center of the solar system, the Earth moves around the sun. Scientists said, well okay, Nicolaus, if that's true we ought to be able to detect the motion of the Earth around the sun. And so this slide here illustrates a concept known as stellar parallax. And astronomers said, if the Earth is moving and we look at a prominent star, let's say, Sirius -- well I know I'm in Manhattan so you guys can't see the stars, but imagine you're out in the country, imagine you chose that rural life -- and we look at a star in December, we see that star against the backdrop of distant stars. If we now make the same observation six months later when the Earth has moved to this position in June, we look at that same star and we see it against a different backdrop. That difference, that angular difference, is the stellar parallax. So this is a prediction that the Copernican model makes. Astronomers looked for the stellar parallax and they found nothing, nothing at all. And many people argued that this proved that the Copernican model was false.
So what happened? Well, in hindsight we can say that astronomers were making two auxiliary hypotheses, both of which we would now say were incorrect. The first was an assumption about the size of the Earth's orbit. Astronomers were assuming that the Earth's orbit was large relative to the distance to the stars. Today we would draw the picture more like this, this comes from NASA, and you see the Earth's orbit is actually quite small. In fact, it's actually much smaller even than shown here. The stellar parallax therefore, is very small and actually very hard to detect.
And that leads to the second reason why the prediction didn't work, because scientists were also assuming that the telescopes they had were sensitive enough to detect the parallax. And that turned out not to be true. It wasn't until the 19th century that scientists were able to detect the stellar parallax.
So, there's a third problem as well. The third problem is simply a factual problem, that a lot of science doesn't fit the textbook model. A lot of science isn't deductive at all, it's actually inductive. And by that we mean that scientists don't necessarily start with theories and hypotheses, often they just start with observations of stuff going on in the world. And the most famous example of that is one of the most famous scientists who ever lived, Charles Darwin. When Darwin went out as a young man on the voyage of the Beagle, he didn't have a hypothesis, he didn't have a theory. He just knew that he wanted to have a career as a scientist and he started to collect data. Mainly he knew that he hated medicine because the sight of blood made him sick so he had to have an alternative career path. So he started collecting data. And he collected many things, including his famous finches. When he collected these finches, he threw them in a bag and he had no idea what they meant. Many years later back in London, Darwin looked at his data again and began to develop an explanation, and that explanation was the theory of natural selection.
Besides inductive science, scientists also often participate in modeling. One of the things scientists want to do in life is to explain the causes of things. And how do we do that? Well,one way you can do it is to build a model that tests an idea.
So this is a picture of Henry Cadell, who was a Scottish geologist in the 19th century. You can tell he's Scottish because he's wearing a deerstalker cap and Wellington boots. (Laughter) And Cadell wanted to answer the question, how are mountains formed? And one of the things he had observed is that if you look at mountains like the Appalachians, you often find that the rocks in them are folded, and they're folded in a particular way, which suggested to him that they were actually being compressed from the side. And this idea would later play a major role in discussions of continental drift. So he built this model, this crazy contraption with levers and wood, and here's his wheelbarrow, buckets, a big sledgehammer. I don't know why he's got the Wellington boots. Maybe it's going to rain. And he created this physical model in order to demonstrate that you could, in fact, create patterns in rocks, or at least, in this case, in mud, that looked a lot like mountains if you compressed them from the side. So it was an argument about the cause of mountains.
Nowadays, most scientists prefer to work inside, so they don't build physical models so much as to make computer simulations. But a computer simulation is a kind of a model. It's a model that's made with mathematics, and like the physical models of the 19th century, it's very important for thinking about causes. So one of the big questions to do with climate change, we have tremendous amounts of evidence that the Earth is warming up. This slide here, the black line shows the measurements that scientists have taken for the last 150 years showing that the Earth's temperature has steadily increased, and you can see in particular that in the last 50 years there's been this dramatic increase of nearly one degree centigrade, or almost two degrees Fahrenheit.
So what, though, is driving that change? How can we know what's causing the observed warming? Well, scientists can model it using a computer simulation. So this diagram illustrates a computer simulation that has looked at all the different factors that we know can influence the Earth's climate, so sulfate particles from air pollution, volcanic dust from volcanic eruptions, changes in solar radiation, and, of course, greenhouse gases. And they asked the question, what set of variables put into a model will reproduce what we actually see in real life? So here is the real life in black. Here's the model in this light gray, and the answer is a model that includes, it's the answer E on that SAT, all of the above. The only way you can reproduce the observed temperature measurements is with all of these things put together, including greenhouse gases, and in particular you can see that the increase in greenhouse gases tracks this very dramatic increase in temperature over the last 50 years. And so this is why climate scientists say it's not just that we know that climate change is happening, we know that greenhouse gases are a major part of the reason why.
So now because there all these different things that scientists do, the philosopher Paul Feyerabend famously said, "The only principle in science that doesn't inhibit progress is: anything goes." Now this quotation has often been taken out of context, because Feyerabend was not actually saying that in science anything goes. What he was saying was, actually the full quotation is, "If you press me to say what is the method of science, I would have to say: anything goes." What he was trying to say is that scientists do a lot of different things. Scientists are creative.
But then this pushes the question back: If scientists don't use a single method, then how do they decide what's right and what's wrong? And who judges? And the answer is, scientists judge, and they judge by judging evidence. Scientists collect evidence in many different ways, but however they collect it, they have to subject it to scrutiny. And this led the sociologist Robert Merton to focus on this question of how scientists scrutinize data and evidence, and he said they do it in a way he called "organized skepticism." And by that he meant it's organized because they do it collectively, they do it as a group, and skepticism, because they do it from a position of distrust. That is to say, the burden of proof is on the person with a novel claim. And in this sense, science is intrinsically conservative. It's quite hard to persuade the scientific community to say, "Yes, we know something, this is true." So despite the popularity of the concept of paradigm shifts, what we find is that actually, really major changes in scientific thinking are relatively rare in the history of science.
So finally that brings us to one more idea: If scientists judge evidence collectively, this has led historians to focus on the question of consensus, and to say that at the end of the day, what science is, what scientific knowledge is, is the consensus of the scientific experts who through this process of organized scrutiny, collective scrutiny, have judged the evidence and come to a conclusion about it, either yea or nay.
So we can think of scientific knowledge as a consensus of experts. We can also think of science as being a kind of a jury, except it's a very special kind of jury. It's not a jury of your peers, it's a jury of geeks. It's a jury of men and women with Ph.D.s, and unlike a conventional jury, which has only two choices, guilty or not guilty, the scientific jury actually has a number of choices. Scientists can say yes, something's true. Scientists can say no, it's false. Or, they can say, well it might be true but we need to work more and collect more evidence. Or, they can say it might be true, but we don't know how to answer the question and we're going to put it aside and maybe we'll come back to it later. That's what scientists call "intractable."
But this leads us to one final problem: If science is what scientists say it is, then isn't that just an appeal to authority? And weren't we all taught in school that the appeal to authority is a logical fallacy? Well, here's the paradox of modern science, the paradox of the conclusion I think historians and philosophers and sociologists have come to, that actually science is the appeal to authority, but it's not the authority of the individual, no matter how smart that individual is, like Plato or Socrates or Einstein. It's the authority of the collective community. You can think of it is a kind of wisdom of the crowd, but a very special kind of crowd. Science does appeal to authority, but it's not based on any individual, no matter how smart that individual may be. It's based on the collective wisdom, the collective knowledge, the collective work, of all of the scientists who have worked on a particular problem. Scientists have a kind of culture of collective distrust, this "show me" culture, illustrated by this nice woman here showing her colleagues her evidence. Of course, these people don't really look like scientists, because they're much too happy. (Laughter)
Okay, so that brings me to my final point. Most of us get up in the morning. Most of us trust our cars. Well, see, now I'm thinking, I'm in Manhattan, this is a bad analogy, but most Americans who don't live in Manhattan get up in the morning and get in their cars and turn on that ignition, and their cars work, and they work incredibly well. The modern automobile hardly ever breaks down.
So why is that? Why do cars work so well? It's not because of the genius of Henry Ford or Karl Benz or even Elon Musk. It's because the modern automobile is the product of more than 100 years of work by hundreds and thousands and tens of thousands of people. The modern automobile is the product of the collected work and wisdom and experience of every man and woman who has ever worked on a car, and the reliability of the technology is the result of that accumulated effort. We benefit not just from the genius of Benz and Ford and Musk but from the collective intelligence and hard work of all of the people who have worked on the modern car. And the same is true of science, only science is even older. Our basis for trust in science is actually the same as our basis in trust in technology, and the same as our basis for trust in anything, namely, experience.
But it shouldn't be blind trust any more than we would have blind trust in anything. Our trust in science, like science itself, should be based on evidence, and that means that scientists have to become better communicators. They have to explain to us not just what they know but how they know it, and it means that we have to become better listeners.
Thank you very much.
(Applause)
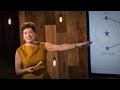
私たちは日々 気候変動やワクチンの安全性などの 問題に直面し 答えを出さなければならないわけですが その答えは科学的な情報に かなり依存しています 科学者は世界が温暖化していると 言いますし ワクチンが安全だとも言いますが 彼らが正しいと どうして分かるのでしょう なぜ科学を信用すべきなのでしょうか? 実際 科学を信用しない人も大勢います 世論調査が絶えず示すのは アメリカ国民のうち かなりの割合の人々が 人類の活動のせいで 温暖化が起きているとは思わず 自然淘汰による進化を信じず ワクチンの安全性を疑っています
なぜ私たちは 科学を信じるべきなのでしょうか 科学者は科学を信じる信じないで 語ることを好みません 事実 彼らは科学と信仰を 正反対のものと考え 信じるというのは 信仰の範疇のものだと言うでしょう 信仰は科学とはかけ離れた まったく別のものです 科学者に言わせれば 宗教は信仰に基づいているか パスカルの賭けの論法に 基づいているのです ブレーズ・パスカルは 17世紀の数学者で 神を信じるべきかどうかという問題に 科学的論拠を使おうとした人です 彼の賭けはこんな感じです もし神が存在しないのに 私はその存在を信じることにしても あまり損はない 日曜日の数時間が取られる程度 (笑) でも もし神が存在するのに 私がそれを信じなければ 非常にまずいことになる だからパスカルが言うには 「神は信じた方がいい」 大学時代のある先生の言葉を借りれば 「彼は信仰の手すりを求めた」 彼は科学と合理主義を捨てて 論理を超えた賭けに出たわけです
ところが実際のところ 大抵の人にとって ほとんどの科学的主張は 論理を超えた賭けです ほとんどの場合 私たちは自分で 科学的主張を評価することはできません 実は ほとんどの科学者にとっても 自分の専門以外の分野では そうなのです 考えてみてください 地質学者はワクチンが安全か 答えられません ほとんどの化学者は 進化論の専門家ではありません 物理学者には 他の科学者が断言しようとも タバコがガンの原因かどうか わかりません 当の科学者たちでさえ 専門外に関しては 論理を超えて 賭けに出なければならないなら なぜ科学者は他の科学者の主張を 聞き入れるのでしょうか なぜ彼らは お互いの主張を信じるのでしょう そして私たちはその主張を 信じるべきなのでしょうか
私の意見はイエス 信じるべきです ただし大抵の人が考えるのとは 理由が違います 大抵の人は学校で 科学を信じるべき 理由となるのは 科学的手法だと教わりました 科学者はある手法に従い この手法が 彼らの主張が真であることを 保証するのだと教わりました 大抵の人が学校で教わった ― 教科書どおりの手法は 仮説に基づく演繹法です 標準的な教科書どおりのモデルによると 科学者は仮説を立て その仮説の論理的結論を推論し 実際に試して こう問います 「さあ この結論は合っているか?」 「自然界で この現象が観測できるか?」 そして合っていれば科学者はこう言います 「よし 仮説は正しいと立証された」
まさにこれを行った科学者の 有名な例が科学史上にたくさんあります 最も有名な例は アルベルト・アインシュタインです アインシュタインが 一般相対性理論を構築した時 彼の理論における結論の一つに 四次元時空は単なる カラッポの空間ではなく そこには布があって その布が太陽のような ― 大質量の物体によって たわむというのが ありました つまり この理論が正しければ 光は 太陽の傍を通過する時 その付近で曲げられることになります それはかなり衝撃的な予測でした 科学者による確認が可能になるまでに 数年かかりましたが 1919年に確認し なんと理論は正しいと実証されました 太陽の近傍を通る光は 実際に曲がるのです これが理論を立証する決め手となりました 例の斬新な考えが正しいという 証拠と見なされ 世界中の多くの新聞が 大々的に扱いました
さて この理論あるいはモデルは 演繹的・法則的モデルと 言われたりします それは主に学者が 事をややこしくするのを好むせいですが 理想的な場合 法則が関わるせいでもあります つまり法則が関係しているということです 理想的な場合 仮説はただの思いつきではなく 理想的には自然の法則なのです 自然の法則であることが なぜ重要なのでしょう もしそれが自然の法則なら 絶対だからです それがもし法則なら常に いつでもどこでも どんな条件下であれ 真なのです どなたでも有名な法則を 1つはご存じです アインシュタインの有名な関係式 E=mc2 エネルギーと質量が どんな関係か示してくれる式です そしてその関係は どんな時も必ず成り立ちます
ところが このモデルには いくつかの問題があります 主な問題は それが間違っているということ 真ではないのです(笑) 間違いだという根拠を3つお話しします まずは論理上の問題 後件肯定の虚偽という問題です これもまた凝った学術的な言い方ですが 要は 誤った理論からでも 真の予測は可能だと言うことです つまり予測が真であるからと言って その理論が正しいという 論理的な証明にはなりません これについても科学史に良い例があります こちらはプトレマイオスの宇宙の図です 地球が宇宙の中心にあり 太陽と惑星がその周りを回っています プトレマイオスの説は何世紀もの間 非常に聡明な多くの人々に 信じられていました 何故でしょうか? 答えは その説から真の予測が 数多くできたからです プトレマイオスの体系のおかげで 天文学者は惑星運動を 正確に予測できました 実際 当初の予測は 現在の私たちが真と考える地動説より 正確なものでした これが教科書モデルの 問題点の1つ目です 2つ目は実務上の問題 補助仮説の問題です 補助仮説とは 科学者が持つ前提のことですが 彼ら自身も意識していないかもしれません これについて重要な例を 最終的に天動説の座を引き継いだ 地動説からご紹介します ニコラウス・コペルニクスが 地球は宇宙の中心ではなく 太陽が太陽系の中心で 地球は太陽の周りを移動している と言った時 科学者たちは こう言いました 「いいかいニコラウス それがもし本当なら 太陽の周りを回る地球の運動を 検出できることになる」 こちらは年周視差として知られる 概念の説明です 天文学者は言いました もし地球が動いているなら よく見える星 たとえばシリウスを見て ― まぁマンハッタンでは 星は見えませんけどね 田舎にいると思ってください 田舎暮らしをして ― 12月に ある星を見ると その星の後ろには 遠くの星が見えます もし私たちが同じ観察を半年後に行うと 6月に地球は この位置に動いていますから 同じ星を見ると その背景が違っているわけです この角度の違いが年周視差です こちらは地動説による予測です 天文学者たちは年周視差を探しましたが まったく何も見つかりませんでした これにより多くの人が地動説は 誤りだと証明されたと主張しました
何故そうなったのでしょうか? 今の私たちには 当時の天文学者が 2つの補助仮説を立てていて そのどちらも不適当だったとわかります 1つは地球の軌道の大きさに関する前提 天文学者は他の星との距離から算出し 地球の軌道を大きく見積もっていました 今日 私たちが描くのはこんな図です NASAの画像です 地球の軌道はかなり小さいでしょう 実は ここに描かれているよりも ずっと小さいんですよ そのため年周視差は 非常に小さく 検出するのは非常に困難なのです
このことは予測どおり行かなかった ― 理由の2つ目と関連してきます 科学者は自分たちの望遠鏡が 視差を検出できるほど高感度だと 思っていたのです そうではありませんでした 科学者が年周視差を検出するのは 19世紀になるまで 不可能でした
さて問題の3つ目です 3つ目の問題は事実に関する問題で 科学の多くが教科書モデルに 該当しないということです 科学の多くは決して演繹的ではなく 実際には帰納的なのです つまり科学者は必ずしも 理論や仮説から出発するわけではなく 世界で起きていることの観察から 出発することも多々あるのです この例として最も有名なのは かの有名な科学者 チャールズ・ダーウィンです 若き日のダーウィンが ビーグル号に乗船して旅に出た時 彼は仮説も理論も持っていませんでした ただ科学者としての経歴を持ちたい その一心で 彼はデータを集め始めました なにしろ彼は医学をやるのが嫌でした 血を見ると気分が悪くなるからです だから別の進路が必要だったのです それでデータ収集を始めました あの有名なフィンチを含め 様々なものを集めました 採集の際 彼はフィンチを袋に放り込み その意味も認識していませんでした 何年も後 ロンドンで ダーウィンはデータを見直し 解釈を見出し始めました その解釈が自然選択説です
帰納的な科学に加え 科学者がよく使う手法に モデリングがあります 科学者が人生で実現したいことの一つに 原因の説明があります どうやるのでしょうか? 方法の一つは アイディアを試すための モデルを作ることです
こちらの写真はヘンリー・キャデル 19世紀のスコットランド人地質学者です スコットランド人だというのは 鹿撃ち帽にウェリントン・ブーツで 一目瞭然です (笑) キャデルが解こうとした問題は 「山はどうやって出来るのか?」でした 彼は気づきました アパラチアのような山脈を見ると 岩が褶曲(しゅうきょく)しているのを 見かけますよね 岩の独特な曲がり方から 彼はピンと来ました 岩は側面から圧迫を受けていたのです そして このアイディアは後に 大陸移動の議論で 重要な役目を果たしました 彼はこれをモデル化し テコと木材で 奇抜な仕掛けを作りました 手押し車や バケツや大きなゲンノウもありますね ウェリントン・ブーツの理由は不明です 雨だったのかしらね 彼がこの物理的モデルを作ったのは 側面から圧力をかけると 岩や この場合で言うと泥に 山にかなり似た模様が作り出せると 実証するのが目的でした それは山ができる原因についての 主張でした
最近の科学者は屋内での仕事を好むので 物理的モデルはあまり作りませんが コンピュータ・シミュレーションはします コンピュータ・シミュレーションは モデルの一種です それは数学によるモデルで 19世紀の物理モデル同様 原因について思考するために 非常に重要です 気候変動に関する大問題の一つを 挙げましょう 地球の温暖化については 膨大な証拠があります こちらのスライドの黒い線は ここ150年間の 科学者による測定結果で 地球の温度の 着実な上昇を示しています 特に最近50年で このように劇的に 上昇しているのがわかります 摂氏1度近く あるいは華氏2度ほどの上昇です
でも 何がその変化を促しているのでしょう 観測された温暖化の原因が何か どうしたらわかるのでしょう 科学者はシミュレーションを使って それをモデル化できるのです こちらはコンピュータ・シミュレーションの 説明図です 地球の気候に影響を与え得る 様々な因子を残らず調べています 大気汚染から出る硫酸塩粒子 火山の噴火から出る火山灰 太陽放射の変動 当然 温室効果ガスも入っています そして科学者は調べます どの変数の組み合わせを モデルに入れると 現実に起きていることを再現できるか この黒い線が現実です モデルはこの薄いグレーの線 そして出た答えは 学力試験の選択肢で お馴染みの 「上記のすべて」を含むモデルです 計測された温度を 再現するためには 温室効果ガスを含む全ての因子を 取り込むより他にないのです とりわけ ご覧のとおり 温室効果ガスの増加が ここ50年の間の この非常に劇的な気温上昇の 動きを追っています ですから これを根拠に気候学者は 気候変動が起きているだけではなく 温室効果ガスがその理由の 主要な部分を 占めているのは明らかだと 言えるわけです
さて このように科学者が違う研究を 行っていることから 哲学者ポール・ファイヤアーベントは ご存知の通り こう言いました 「『何でもあり』の精神こそが 科学の進歩を妨げない 唯一の原理である」 実はこれは言葉尻を取られていて ファイヤアーベントは 科学では「何でもありだ」とは 言っていないのです 実際に言った 全文はこうです 「科学の手法とは何か 答えろと迫られたら 『何でもありだ』と言わざるを得ない」 彼が言おうとしたのは 科学者は様々な異なることをしており 創造性豊かだということです
しかし ここであの疑問が戻ってきます 科学者の使う手法がバラバラなら 何が正しく何が間違っているか どうやって決めるのでしょう 誰が判断するのでしょう 答えは 科学者が判断するのです その判断は証拠の判断によります 科学者は様々な異なる方法で 証拠を集めますが それが どんな方法であれ 証拠を検査にかけなければなりません 社会学者ロバート・マートンは 科学者がどうやってデータや 証拠を検査するかという 問題に着目し その方法を 「組織的懐疑主義」と呼びました 彼が組織化されていると考えたのは 科学者たちが共同で 集団として検査を行うからで 懐疑主義だと考えたのは 科学者がそれを 不信をベースに行うからです すなわち 立証責任を負うのは 新しい主張を持ち込んだ その人物です この意味で 科学は本質的に保守的です 科学界を説得し 「よし これは明らかに真だ」と 言わせるのは 非常に厳しいことです だからパラダイム シフトの概念が 支持を集めていようとも 実際のところ 科学的思考に本当に大幅な 変化が起きた例は 科学史上 比較的まれです
このことは いよいよ私たちを 次の考えへと導きます 科学者が集団で証拠を判断することから 歴史学者は合意の問題に 注目してきました 歴史学者の最終的な結論は こうです 科学というもの 科学的知見というものは 組織的で集団的な 検査のプロセスを通っており それは証拠を判断し マルかバツかの 断定をした科学の専門家たちの 総意であるということです
つまり科学的知見は 専門家の総意だと考えられます 科学とは陪審のようなものだと 考えることもできます かなり特殊な陪審ですけどね あまり身近にはいないタイプの オタクの陪審です 博士号を持つ人たちの陪審です そして有罪か無罪か 二者択一の 通常の陪審と違って 科学の陪審には 選択肢がいろいろあります 科学者は「Yes 真である」 と言うこともあれば 「No 偽である」と言うこともあります あるいは「真の可能性はあるが ― もっと研究を重ね 証拠を積み上げる必要がある」とか 「真の可能性はあるが ― 答えようがないので ひとまず保留にして 後々また考えよう」とか言うこともあります これは科学者が 「解決困難」と呼ぶものです
しかしこれが最後の問題につながります 科学が 科学者の意見で 成立しているのなら 単なる権威への訴えかけでは ないのでしょうか 私たちは学校で 権威への訴えは論理上の誤謬だと 教わったのではないでしょうか ここに現代科学の矛盾があります 私が思うに 歴史学者や哲学者や 社会学者が至った ― 科学は権威への訴えだという 結論の矛盾です ただし権威と言っても 特定の人物のことではありません プラトンやソクラテスや アインシュタインのように どんなに頭脳明晰でも ある個人のことではないのです 権威とは科学界全体のことです ある種の「集団の知恵」だと 思えばいいです 非常に特殊な集団ですけどね 科学は権威に訴えかけますが 基準は特定の人物ではありません どんなに頭脳明晰だとしてもです 基準となるのは ある問題について 研究してきた全ての科学者の集団的英知 集団的知見 集合体としての研究成果です 科学者には ある種の 集団的不信の文化があります 「証明してみろ」の文化です こちらの素敵な女性が良い例です 仲間に自分の見つけた証拠を 見せています 勿論 この人たちは科学者にしては ニコニコしすぎですね (笑)
さて では私の最後の論点です 大抵の人は朝起きて 自分の車を信頼しています ここはマンハッタンですから 例えが悪いですけど マンハッタン以外に住む アメリカ人のほとんどは 朝起きて車に乗ります エンジンをかければ車は動きます それも非常によく動きます 現代の車はめったに故障しません
なぜ車はそんなにうまく動くのでしょう ヘンリー・フォードやカール・ベンツや イーロン・マスクらの才能のためでは ありません その理由は現代の車が 百年以上に渡る 何百 何千 何万もの 人々の仕事の 積み重ねだからです 現代の車は 車に関わる仕事をした すべての人の 集合的な研究と知恵と経験の 成果であり テクノロジーの信頼性は 蓄積された努力の結晶なのです 私たちが恩恵を受けているのは ベンツやフォードやマスクらの 才能だけでなく 現代の車に関わった すべての人たちの 集団的な知と勤勉の おかげなのです 科学も同じです ただし科学は車より歴史が長いですが 私たちの科学を信頼する根拠は テクノロジーを信頼する根拠と同じで 対象が何であれ 信頼するときの根拠と同じです すなわち経験がモノを言うのです
しかし盲目的な信頼はダメです 何事においても鵜呑みはいけません 科学自体がそうであるように 私たちの科学に対する信頼も 証拠に基づいていなければなりません だから科学者は もっと上手に 伝えるようにしなければなりません 科学者は私たちに結果だけではなく その過程をも 説明しなければなりません そして私たちはもっと上手に 聞けるようにならなければなりません
ありがとうございました
(拍手)
品詞分類
- 主語
- 動詞
- 助動詞
- 準動詞
- 関係詞等
TED 日本語
TED Talks
関連動画
十代の科学者が考えた、傷の治癒を促す発明アヌシュカ・ナイクナーレ
2018.06.19自分の声が嫌だと感じるのはなぜかレベッカ・クラインバーガー
2018.05.24若い発明家の発泡スチロールリサイクル計画アシュトン・コーファー
2017.04.18においの「自撮り」やその他の合成生物学的な実験アニー・リュー
2017.03.17物理学の未解決問題をいかに探求するかジェームズ・ビーチャム
2017.01.20世界を理解する奥義としての数学ロジャー・アントンセン
2016.12.13機械に奪われる仕事 ― そして残る仕事アンソニー・ゴールドブルーム
2016.08.31次なる科学の大発見とは?エリック・ヘイゼルティン
2016.08.18数学の何がそれほど魅惑的なのかセドリック・ヴィラニ
2016.06.28飛行機内の病原菌の動き方と対処法レイモンド・ワン
2016.01.11科学を良い方向に進める知られざる頭脳ジャダイダ・アイスラー
2016.01.07量子生物学は生命の最大の謎を解明するか?ジム・アルカリリ
2015.09.16バスケットボールの激しい動きの背後にある数学ラジブ・マヒシュワラン
2015.07.06笑わせ、そして考えさせる科学賞マーク・エイブラハムズ
2014.10.24知能の方程式アレックス・ウィスナー=グロス
2014.02.06子犬たち!さあ、複合性理論だよ!ニコラ・ペロニー
2014.01.30
洋楽 おすすめ
RECOMMENDS
洋楽歌詞
ダイナマイトビーティーエス
洋楽最新ヒット2020.08.20ディス・イズ・ミーグレイテスト・ショーマン・キャスト
洋楽人気動画2018.01.11グッド・ライフGイージー、ケラーニ
洋楽人気動画2017.01.27ホワット・ドゥ・ユー・ミーン?ジャスティン・ビーバー
洋楽人気動画2015.08.28ファイト・ソングレイチェル・プラッテン
洋楽人気動画2015.05.19ラヴ・ミー・ライク・ユー・ドゥエリー・ゴールディング
洋楽人気動画2015.01.22アップタウン・ファンクブルーノ・マーズ、マーク・ロンソン
洋楽人気動画2014.11.20ブレイク・フリーアリアナ・グランデ
洋楽人気動画2014.08.12ハッピーファレル・ウィリアムス
ポップス2014.01.08カウンティング・スターズワンリパブリック
ロック2013.05.31ア・サウザンド・イヤーズクリスティーナ・ペリー
洋楽人気動画2011.10.26ユー・レイズ・ミー・アップケルティック・ウーマン
洋楽人気動画2008.05.30ルーズ・ユアセルフエミネム
洋楽人気動画2008.02.21ドント・ノー・ホワイノラ・ジョーンズ
洋楽人気動画2008.02.15オンリー・タイムエンヤ
洋楽人気動画2007.10.03ミス・ア・シングエアロスミス
ロック2007.08.18タイム・トゥ・セイ・グッバイサラ・ブライトマン
洋楽人気動画2007.06.08シェイプ・オブ・マイ・ハートスティング
洋楽人気動画2007.03.18ウィ・アー・ザ・ワールド(U.S.A. フォー・アフリカ)マイケル・ジャクソン
洋楽人気動画2006.05.14ホテル・カリフォルニアイーグルス
ロック2005.07.06