
TED日本語
TED Talks(英語 日本語字幕付き動画)
TED日本語 - エド・ボイデン: 脳の見えない秘密を調べる新しい方法
TED Talks
脳の見えない秘密を調べる新しい方法
A new way to study the brain's invisible secrets
エド・ボイデン
Ed Boyden
内容
神経工学者のエド・ボイデンは、私達の脳の小さな生体分子がどのように感情、思考、感覚を生み出すのか知ろうとしています。また、てんかんやアルツハイマー病のような脳の疾患を引き起こす生体分子の変化を突き止めたいとも願っています。「見えない構造を顕微鏡で拡大するより、脳組織を物理的に拡大したら見やすくなるのでは?」と彼は考えました。紙おむつの吸収材に使われるポリマーが、なぜ私達の脳をもっと良く理解する為のカギとなり得るのか、知りたいと思いませんか。
字幕
SCRIPT
Script
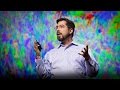
Hello, everybody. I brought with me today a baby diaper. You'll see why in a second. Baby diapers have interesting properties. They can swell enormously when you add water to them, an experiment done by millions of kids every day.
(Laughter)
But the reason why is that they're designed in a very clever way. They're made out of a thing called a swellable material. It's a special kind of material that, when you add water, it will swell up enormously, maybe a thousand times in volume. And this is a very useful, industrial kind of polymer. But what we're trying to do in my group at MIT is to figure out if we can do something similar to the brain. Can we make it bigger, big enough that you can peer inside and see all the tiny building blocks, the biomolecules, how they're organized in three dimensions, the structure, the ground truth structure of the brain, if you will? If we could get that, maybe we could have a better understanding of how the brain is organized to yield thoughts and emotions and actions and sensations. Maybe we could try to pinpoint the exact changes in the brain that result in diseases, diseases like Alzheimer's and epilepsy and Parkinson's, for which there are few treatments, much less cures, and for which, very often, we don't know the cause or the origins and what's really causing them to occur. Now, our group at MIT is trying to take a different point of view from the way neuroscience has been done over the last hundred years. We're designers. We're inventors. We're trying to figure out how to build technologies that let us look at and repair the brain. And the reason is, the brain is incredibly, incredibly complicated.
So what we've learned over the first century of neuroscience is that the brain is a very complicated network, made out of very specialized cells called neurons with very complex geometries, and electrical currents will flow through these complexly shaped neurons. Furthermore, neurons are connected in networks. They're connected by little junctions called synapses that exchange chemicals and allow the neurons to talk to each other. The density of the brain is incredible. In a cubic millimeter of your brain, there are about 100,000 of these neurons and maybe a billion of those connections. But it's worse. So, if you could zoom in to a neuron, and, of course, this is just our artist's rendition of it. What you would see are thousands and thousands of kinds of biomolecules, little nanoscale machines organized in complex, 3D patterns, and together they mediate those electrical pulses, those chemical exchanges that allow neurons to work together to generate things like thoughts and feelings and so forth.
Now, we don't know how the neurons in the brain are organized to form networks, and we don't know how the biomolecules are organized within neurons to form these complex, organized machines. If we really want to understand this, we're going to need new technologies. But if we could get such maps, if we could look at the organization of molecules and neurons and neurons and networks, maybe we could really understand how the brain conducts information from sensory regions, mixes it with emotion and feeling, and generates our decisions and actions. Maybe we could pinpoint the exact set of molecular changes that occur in a brain disorder. And once we know how those molecules have changed, whether they've increased in number or changed in pattern, we could use those as targets for new drugs, for new ways of delivering energy into the brain in order to repair the brain computations that are afflicted in patients who suffer from brain disorders.
We've all seen lots of different technologies over the last century to try to confront this. I think we've all seen brain scans taken using MRI machines. These, of course, have the great power that they are noninvasive, they can be used on living human subjects. But also, they're spatially crude. Each of these blobs that you see, or voxels, as they're called, can contain millions and millions of neurons. So it's not at the level of resolution where it can pinpoint the molecular changes that occur or the changes in the wiring of these networks that contributes to our ability to be conscious and powerful beings.
At the other extreme, you have microscopes. Microscopes, of course, will use light to look at little tiny things. For centuries, they've been used to look at things like bacteria. For neuroscience, microscopes are actually how neurons were discovered in the first place, about 130 years ago. But light is fundamentally limited. You can't see individual molecules with a regular old microscope. You can't look at these tiny connections. So if we want to make our ability to see the brain more powerful, to get down to the ground truth structure, we're going to need to have even better technologies.
My group, a couple years ago, started thinking: Why don't we do the opposite? If it's so darn complicated to zoom in to the brain, why can't we make the brain bigger? It initially started with two grad students in my group, Fei Chen and Paul Tillberg. Now many others in my group are helping with this process. We decided to try to figure out if we could take polymers, like the stuff in the baby diaper, and install it physically within the brain. If we could do it just right, and you add water, you can potentially blow the brain up to where you could distinguish those tiny biomolecules from each other. You would see those connections and get maps of the brain. This could potentially be quite dramatic.
We brought a little demo here. We got some purified baby diaper material. It's much easier just to buy it off the Internet than to extract the few grains that actually occur in these diapers. I'm going to put just one teaspoon here of this purified polymer. And here we have some water. What we're going to do is see if this teaspoon of the baby diaper material can increase in size. You're going to see it increase in volume by about a thousandfold before your very eyes. I could pour much more of this in there, but I think you've got the idea that this is a very, very interesting molecule, and if can use it in the right way, we might be able to really zoom in on the brain in a way that you can't do with past technologies.
OK. So a little bit of chemistry now. What's going on in the baby diaper polymer? If you could zoom in, it might look something like what you see on the screen. Polymers are chains of atoms arranged in long, thin lines. The chains are very tiny, about the width of a biomolecule, and these polymers are really dense. They're separated by distances that are around the size of a biomolecule. This is very good because we could potentially move everything apart in the brain. If we add water, what will happen is, this swellable material is going to absorb the water, the polymer chains will move apart from each other, and the entire material is going to become bigger. And because these chains are so tiny and spaced by biomolecular distances, we could potentially blow up the brain and make it big enough to see.
Here's the mystery, then: How do we actually make these polymer chains inside the brain so we can move all the biomolecules apart? If we could do that, maybe we could get ground truth maps of the brain. We could look at the wiring. We can peer inside and see the molecules within. To explain this, we made some animations where we actually look at, in these artist renderings, what biomolecules might look like and how we might separate them. Step one: what we'd have to do, first of all, is attach every biomolecule, shown in brown here, to a little anchor, a little handle. We need to pull the molecules of the brain apart from each other, and to do that, we need to have a little handle that allows those polymers to bind to them and to exert their force.
Now, if you just take baby diaper polymer and dump it on the brain, obviously, it's going to sit there on top. So we need to find a way to make the polymers inside. And this is where we're really lucky. It turns out, you can get the building blocks, monomers, as they're called, and if you let them go into the brain and then trigger the chemical reactions, you can get them to form those long chains, right there inside the brain tissue. They're going to wind their way around biomolecules and between biomolecules, forming those complex webs that will allow you, eventually, to pull apart the molecules from each other. And every time one of those little handles is around, the polymer will bind to the handle, and that's exactly what we need in order to pull the molecules apart from each other.
All right, the moment of truth. We have to treat this specimen with a chemical to kind of loosen up all the molecules from each other, and then, when we add water, that swellable material is going to start absorbing the water, the polymer chains will move apart, but now, the biomolecules will come along for the ride. And much like drawing a picture on a balloon, and then you blow up the balloon, the image is the same, but the ink particles have moved away from each other. And that's what we've been able to do now, but in three dimensions.
There's one last trick. As you can see here, we've color-coded all the biomolecules brown. That's because they all kind of look the same. Biomolecules are made out of the same atoms, but just in different orders. So we need one last thing in order to make them visible. We have to bring in little tags, with glowing dyes that will distinguish them. So one kind of biomolecule might get a blue color. Another kind of biomolecule might get a red color. And so forth. And that's the final step. Now we can look at something like a brain and look at the individual molecules, because we've moved them far apart enough from each other that we can tell them apart.
So the hope here is that we can make the invisible visible. We can turn things that might seem small and obscure and blow them up until they're like constellations of information about life. Here's an actual video of what it might look like. We have here a little brain in a dish -- a little piece of a brain, actually. We've infused the polymer in, and now we're adding water. What you'll see is that, right before your eyes -- this video is sped up about sixtyfold -- this little piece of brain tissue is going to grow. It can increase by a hundredfold or even more in volume. And the cool part is, because those polymers are so tiny, we're separating biomolecules evenly from each other. It's a smooth expansion. We're not losing the configuration of the information. We're just making it easier to see.
So now we can take actual brain circuitry -- here's a piece of the brain involved with, for example, memory -- and we can zoom in. We can start to actually look at how circuits are configured. Maybe someday we could read out a memory. Maybe we could actually look at how circuits are configured to process emotions, how the actual wiring of our brain is organized in order to make us who we are. And of course, we can pinpoint, hopefully, the actual problems in the brain at a molecular level. What if we could actually look into cells in the brain and figure out, wow, here are the 17 molecules that have altered in this brain tissue that has been undergoing epilepsy or changing in Parkinson's disease or otherwise being altered? If we get that systematic list of things that are going wrong, those become our therapeutic targets. We can build drugs that bind those. We can maybe aim energy at different parts of the brain in order to help people with Parkinson's or epilepsy or other conditions that affect over a billion people around the world.
Now, something interesting has been happening. It turns out that throughout biomedicine, there are other problems that expansion might help with. This is an actual biopsy from a human breast cancer patient. It turns out that if you look at cancers, if you look at the immune system, if you look at aging, if you look at development -- all these processes are involving large-scale biological systems. But of course, the problems begin with those little nanoscale molecules, the machines that make the cells and the organs in our body tick. So what we're trying to do now is to figure out if we can actually use this technology to map the building blocks of life in a wide variety of diseases. Can we actually pinpoint the molecular changes in a tumor so that we can actually go after it in a smart way and deliver drugs that might wipe out exactly the cells that we want to?
You know, a lot of medicine is very high risk. Sometimes, it's even guesswork. My hope is we can actually turn what might be a high-risk moon shot into something that's more reliable. If you think about the original moon shot, where they actually landed on the moon, it was based on solid science. We understood gravity; we understood aerodynamics. We knew how to build rockets. The science risk was under control. It was still a great, great feat of engineering. But in medicine, we don't necessarily have all the laws. Do we have all the laws that are analogous to gravity, that are analogous to aerodynamics? I would argue that with technologies like the kinds I'm talking about today, maybe we can actually derive those. We can map the patterns that occur in living systems, and figure out how to overcome the diseases that plague us.
You know, my wife and I have two young kids, and one of my hopes as a bioengineer is to make life better for them than it currently is for us. And my hope is, if we can turn biology and medicine from these high-risk endeavors that are governed by chance and luck, and make them things that we win by skill and hard work, then that would be a great advance.
Thank you very much.
(Applause)
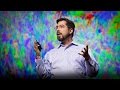
みなさん こんにちは 赤ちゃんの紙おむつを持ってきました 今 その理由がお分かりになります 紙おむつには 興味深い素材が使われています 紙おむつが水分を吸うと 非常に膨れ上がることは 日々何百万人もの赤ちゃんが 実証してくれています
(笑)
その理由というのは 紙おむつが巧妙に考案され 膨潤性のある素材から 作られているからです その特別な素材は 水を加えると 驚く程膨れ上がり 千倍もの容積になります これは有用な工業用ポリマーですが MITの私のグループは 我々の脳にも同じような事が できないかと研究しています 脳をもっと大きく 脳内部を覗ける程大きくして 細かな構成部分の生体分子全てが 3次元的にどのような構成になっているか 脳の実体を見られないだろうかというものです それができれば 我々の脳がどのように 思考 感情 行動 感覚を 生み出しているのか もっと良くわかるでしょうし 脳のどの箇所の変化が 様々な病気を起こしているかを 的確に突き止められるかも知れません アルツハイマー病、てんかん、パーキンソン病などです これらの病気の完治は勿論のこと 治療法はほとんどなく 何が原因で発症するか はっきりとは分かっていません MITの我々の研究グループは 今までとは異なる視点を持ち 過去 100年の神経科学とは 違った方法を試みています 我々はデザイナーでもあり 発明家でもあります 我々が研究しているのは 脳内を見て修復する技術を 作り上げることです そんな技術が必要な理由は 我々の脳は非常に複雑に できているからです
20世紀を通して 我々が神経科学で学んだ事は 脳は非常に複雑なネットワークであり 特殊化した細胞 ニューロンが とても込み入った配置をなし この複雑に構成されたニューロンを通り 電気が流れているということです さらにネットワークを 作っているニューロンは シナプスという小さな結合部で繋がり 化学物質で伝達し合い 互いに情報交換をしています 我々の脳の密度はとても高く 脳の1ミリ立方メートル内に 約十万個のニューロン 十億ものシナプスがあり得るということです それだけではなく 1つのニューロンにズームインしてみると もちろんこれは概念図にすぎませんが ― 何千種もの生体分子が見られ その小さなナノスケールの生体分子が 複雑な3Dの形態で構成され 共に電気のパルスを送り 化学物質を交換し合い ニューロンを恊働させ 思考や感情などを生み出しています
ニューロンがどのように脳内に ネットワークを作っているのか 分かっていません このような生体分子が どのようにニューロン内で組織化され こうした複雑に組織立った機能を 持っているのか分かっていません 本当にこれを理解したいなら 新しいテクノロジーが必要になるでしょう そんな地図を手に入れて 分子とニューロンや ニューロンとネットワークの構成が 見られたとしたら 恐らく我々の脳がどうやって 知覚野からの情報を処理して 感情や感覚とミックスして 我々を決断や行動に移らせるのか 本当に理解できるかも知れません 的確にどの分子グループが 脳の疾患を起こしているか 探し出せるかも知れません 一旦 分子がどのように 変わったかが分かれば 問題の分子が増えようと パターンが変わろうと その情報を新薬の標的として 脳にエネルギーを送り込む 新しい方法の為に使い 脳疾患を持つ患者の脳機能を 修復することができるでしょう
前世紀に生まれた 多種多様なテクノロジーを使い 我々はこの問題に 取り組もうとして来ました 脳画像を撮る MRIはご存知だと思います これは非侵襲的で 高性能であり 生きているヒトに使えますが 空間解像度は低く ボクセルと呼ばれる画素の1つ1つは 何百万ものニューロンを含んでいます それで この解像度のレベルでは 分子レベルの変化や ヒトの意識を制御する高い能力に関わる 神経ネットワークの配線の変化を 突き止めることはできません
その一方 顕微鏡もありますね 勿論 顕微鏡は微細なものを 見るために光を使います 何世紀もバクテリアのようなものを 見るのに使われてきました 神経科学において 神経細胞は顕微鏡を使い発見された というのが始まりで 130年程前のことです しかし顕微鏡で見られるものは 基本的に限られています 普通の顕微鏡では 分子は見分けられません 小さな結合部分を見ることができません 脳をもっと詳細を鮮明に見られるようにして 脳の構造を根本から把握したいならば もっと進んだテクノロジーが 必要になって来るでしょう
数年前 私の研究グループは こう考え始めました 「逆から考えてみよう 脳にズームインするのがそんなに難しいなら 脳を大きくできないだろうか?」 まず2人の大学院生の フェイ・チェンとポール・ティルバーグ と共に始めました 今では私のグループの多くが このプロセスに参加しています 紙おむつに使われている ポリマーが使えるかどうか 脳内に実際埋め込んで 検討してみる事にしました それが きちんと入り 水を加えると こうして脳を膨らますことができ 微細な生体分子の1つ1つが 見分けられます その結合の仕方を見て 脳の地図ができます これはかなり劇的なことになりかねません
その小さなデモを持ってきました ここに精製された紙おむつの素材があります ネットで買った方が オムツに実際に含まれている 少量のポリマーを抽出するよりは ここに小さじ1杯分の 精製されたポリマーが入っています ここに水があります 今から 一さじの紙おむつ素材 ポリマーが 大きくなるかどうか見ます 千倍ほどに膨らむのが 今 見えてきますよ もっと水を入れてもいいのですが これでポリマーが とても興味深い分子だと お分かりになると思います 正しく使えば 今までのテクノロジーでは できないやり方で 本当に脳内にズームイン できるかもしれません
今度は 化学をちょっと 紙おむつのポリマーでは 何が起きているのでしょう? ズームインしてみると スライドのように ポリマーは原子が細長い鎖状になったものです 鎖はとても細く 生体分子の太さくらいです とても密度が高く 鎖間の距離は 生体分子サイズ位です これが とてもいいことなのは 脳内の全てのものを分離させられる かもしれないからです 水を加えると この膨潤性のある材料は水を吸収します ポリマーの鎖同士の距離間が広がり 素材全体が膨張します ポリマーの鎖はとても細く その間隔は生体分子サイズなので 詳しく見られるほど 脳を膨らませることができるでしょう
しかし 問題は 脳の生体分子を分離するため どうやって こんなポリマーの鎖を 脳内につくれるか ということです それができれば 脳地図を作ることが可能となり 神経回路が見られるでしょう こうして中をのぞき 分子を見る事ができます これを説明するため アニメーションを作成しました この概念図で示すのは 生体分子がどのように見えるか そして それらの分離の様子です ステップ1:まず しなくてはならない事は 茶色で示してある 生体分子全てに 小さな取っ手をつけます 脳の分子を1つずつ 離さなくてはならないので それには 小さな取っ手が要ります ポリマーを取っ手と結合させ 力を伝えます
ただ紙おむつのポリマーを 脳の上に置いたら 脳の表面に乗っかっているだけです ポリマーを脳内に入れる方法を 考え出す必要があります ここがとても幸運なところで モノマーと呼ばれる単量体を これに使えるのです それを脳内に入れて 化学反応を引き起こさせ この長いポリマーの鎖を 作る事ができるのです 脳の組織内 その場でです ポリマーは生体分子の周りや 分子間に巻き付き 複雑な網を作り 最後には分子を お互いから引き離します 取っ手の1つがあれば ポリマーは取っ手に結合します まさにこれが必要なことで これが分子をお互いから引き離すのです
実は ここで重要な事が1つ 試料を薬品で処理し 分子の繋がりをほぐさなくてはなりません そうすれば水を加えると 膨潤性のある素材は 水を吸い始めるのです ポリマーの鎖は互いに遠のき始め それと共に生体分子が お互い遠のき合います それは風船に 絵を描くのと似ています そのまま風船を膨らませても 上に描かれたイメージは同じですが 絵のインク分子は 1つ1つ遠のいて行きます それと同じ事が3Dでできたのです
最後に1つの工夫があります ここに見られるように 生体分子は全部茶色に 色分けしてあります どれもみんな似ているからです 生体分子は同じ原子からできていますが ただその並び方が違うのです それで最後にあるものが 分子を見分ける為に必要です 小さな名札です 光る染料で生体分子を 見分けるようにします ある種の生体分子は青で 別の種類は赤という風に 種類別に色を変えます それが最後の段階です これで脳の分子を 1つ1つ見る事ができます なぜなら分子同士が十分離れているので 個々の分子を識別できるからです
ここでは今まで視認できなかったものを 見えるようにすることが目標です 小さくはっきりしないしないものを 膨らませて 生物が持つ情報の集合体が 星座のように見られます これがその様子を示す 実際のビデオです 培養皿に小さな脳が・・・ 脳の一部が少しあります ポリマーが注入されています これに水を加えます 皆様の目の前で起こるのは・・・ このビデオは60倍に 圧縮されています この小さな脳組織が大きくなります 百倍あるいはそれ以上の大きさにもなり得ます これが巧くできているのは ポリマーがとても小さいので 生体分子を等間隔に 分離させている事です スムーズな膨張です 情報の配置関係は失われてはいません ただ見やすくしているだけです
これで実際の脳の神経回路を 見る事ができます 例えば ここに記憶を司る 脳の部分があります ここにズームインして 神経回路がどのように配線されているか 見る事ができます 恐らく将来は記憶を 読み取れるようになるかも 神経回路がどのようになって 感情を生み出しているのか 神経回路の繋がりがどうなって ヒトとしての我々を作っているのか 見られるようになるかも知れません もちろん うまく行けば 的確に 脳内の実際の問題箇所を 分子レベルで突き止められるので 脳内の細胞を実際に見て 「この17個の分子が 脳組織に変化を起こして てんかんの原因となっているんだ」とか パーキンソン病における推移を見られ または修復できるとしたら すごいですね 悪い箇所の系統立ったリストが手に入るなら それが治療の標的となり そこに結合する薬が作られます 脳の特定箇所に 集中的にエネルギー照射をして パーキンソン病やてんかんの患者や その他の脳疾患を持つ 世界中の十億以上の人々を 助けられるかも知れません
面白いことが今起きています 医学において生体分子を 拡大して観察できることが 助けとなる他の問題があるのです これは乳がん患者の生検材料です このように癌や 免疫組織など また加齢や成長過程を見ると これらは全て 生物学的システム全体が関与していますが もちろん それらの問題は 人体が機能する為の細胞や臓器を作る ナノスケールの分子から始まっています そこで今 我々は 様々な病気に広く使えないかと このテクノロジーを使い 生命の構成単位の地図作りに 取り組んでいます 癌に起きる分子の変化を ピンポイントで見つけ 巧い方法でそれを捉えて ちょうどその箇所に薬を運び 除きたい細胞だけをとり除けないか?
ご存知のように薬の多くには 危険を伴い 時には当てずっぽうで投与されます 私の希望は月に行くかのような 危険性の高い無謀な治療を もっと確かなものにすることです もっとも実際にあった月旅行は 月に着陸したので 堅固な科学に基づいていました 重力や 空気力学の理解に基づき 宇宙船は作られました リスクが科学的にコントロールされた それは それは偉大なる 技術の集結でしたが 医療では そんな法則が 必ずしもあるとは言えません 医療に重力や空気力学のような そんな法則がありますか? 今日私が話しているような テクノロジーを使いそんな法則を 導き出せるかもしれません 生体のシステム内で 起きているパターンの地図を作り 我々を悩ます病気を克服する方法を 見つけられます
私と妻には幼い二人の子供たちがいます 生物工学者として私は 子供たちのために今より良い未来をと 願っています そして生物学と医療を 偶然と運にだけに頼る 危険性の高い試みから 日々精進して技能を磨き 可能なものにできるならば それは 大きな進歩となるでしょう
ありがとうございました
(拍手)
品詞分類
- 主語
- 動詞
- 助動詞
- 準動詞
- 関係詞等
TED 日本語
TED Talks
関連動画
うつやPTSDを予防できる新種の薬 | TED Talkレベッカ・ブラックマン
2019.04.17騒音が健康に有害な理由 ― そして私たちにできることマティアス・バスナー
2019.02.26さまよう心を鎮めるには | TED Talkアミシ・ジャー
2018.04.18鬱の友達と心を通わせるにはビル・バーナット
2018.03.23脳に良い変化をもたらす運動の効果ウェンディー・スズキ
おすすめ 12018.03.21物事の「良し悪し」は思い込みに過ぎないヘザー・ラニエ
おすすめ 12018.01.19脳が深い睡眠から更なる恩恵を得る方法ダン・ガーテンバーグ
2018.01.04うつを一人で抱え込まないでニッキー・ウェバー・アレン
2017.10.26長寿の秘訣は周囲の人との交流かもスーザン・ピンカー
2017.09.04脳はどのように美しさを判定するか?アンジャン・チャタジー
2017.08.22脳が「意識された現実」という幻覚を作り出す仕組みアニル・セス
2017.07.18注意を向けた時、脳では何が起きているのかメディ・オディカニ=セイドラー
2017.07.12ティーンエイジャーの登校時間を遅らせるべき理由とはウェンディ・トロクセル
2017.06.09死に直面したとき、人生に生きる価値を与えてくれるのはルーシー・カラニシ
おすすめ 12017.06.07最善の自己と最悪の自己の生物学ロバート・サポルスキー
2017.05.31メンタルヘルスを気遣うのは恥ずかしくなんかないサング・デリ
2017.05.26
洋楽 おすすめ
RECOMMENDS
洋楽歌詞
ダイナマイトビーティーエス
洋楽最新ヒット2020.08.20ディス・イズ・ミーグレイテスト・ショーマン・キャスト
洋楽人気動画2018.01.11グッド・ライフGイージー、ケラーニ
洋楽人気動画2017.01.27ホワット・ドゥ・ユー・ミーン?ジャスティン・ビーバー
洋楽人気動画2015.08.28ファイト・ソングレイチェル・プラッテン
洋楽人気動画2015.05.19ラヴ・ミー・ライク・ユー・ドゥエリー・ゴールディング
洋楽人気動画2015.01.22アップタウン・ファンクブルーノ・マーズ、マーク・ロンソン
洋楽人気動画2014.11.20ブレイク・フリーアリアナ・グランデ
洋楽人気動画2014.08.12ハッピーファレル・ウィリアムス
ポップス2014.01.08カウンティング・スターズワンリパブリック
ロック2013.05.31ア・サウザンド・イヤーズクリスティーナ・ペリー
洋楽人気動画2011.10.26ユー・レイズ・ミー・アップケルティック・ウーマン
洋楽人気動画2008.05.30ルーズ・ユアセルフエミネム
洋楽人気動画2008.02.21ドント・ノー・ホワイノラ・ジョーンズ
洋楽人気動画2008.02.15オンリー・タイムエンヤ
洋楽人気動画2007.10.03ミス・ア・シングエアロスミス
ロック2007.08.18タイム・トゥ・セイ・グッバイサラ・ブライトマン
洋楽人気動画2007.06.08シェイプ・オブ・マイ・ハートスティング
洋楽人気動画2007.03.18ウィ・アー・ザ・ワールド(U.S.A. フォー・アフリカ)マイケル・ジャクソン
洋楽人気動画2006.05.14ホテル・カリフォルニアイーグルス
ロック2005.07.06