
TED日本語
TED Talks(英語 日本語字幕付き動画)
TED日本語 - ジェロ・マイセンブク: 脳の革新
TED Talks
脳の革新
Gero Miesenboeck reengineers a brain
ジェロ・マイセンブク
内容
脳の働きを知るために、多くの科学者はそれぞれのニューロンの活動を記録するという膨大な課題を進めています。ジェロ博士は逆に考え、特定のニューロンを操作しそれらがどのような役割を持っているかを、ハエの光に対する感覚を使った驚くべき方法の数々で解明しようとしています。
字幕
SCRIPT
Script
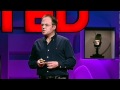
I have a doppelganger.
(Laughter) Dr. Gero is a brilliant but slightly mad scientist in the "Dragonball Z: Android Saga." If you look very carefully, you see that his skull has been replaced with a transparent Plexiglas dome so that the workings of his brain can be observed and also controlled with light. That's exactly what I do -- optical mind control. (Laughter)
But in contrast to my evil twin who lusts after world domination, my motives are not sinister. I control the brain in order to understand how it works. Now wait a minute, you may say, how can you go straight to controlling the brain without understanding it first? Isn't that putting the cart before the horse? Many neuroscientists agree with this view and think that understanding will come from more detailed observation and analysis. They say, "If we could record the activity of our neurons, we would understand the brain." But think for a moment what that means. Even if we could measure what every cell is doing at all times, we would still have to make sense of the recorded activity patterns, and that's so difficult, chances are we'll understand these patterns just as little as the brains that produce them.
Take a look at what brain activity might look like. In this simulation, each black dot is one nerve cell. The dot is visible whenever a cell fires an electrical impulse. There's 10,000 neurons here. So you're looking at roughly one percent of the brain of a cockroach. Your brains are about 100 million times more complicated. Somewhere, in a pattern like this, is you, your perceptions, your emotions, your memories, your plans for the future. But we don't know where, since we don't know how to read the pattern. We don't understand the code used by the brain. To make progress, we need to break the code. But how? An experienced code-breaker will tell you that in order to figure out what the symbols in a code mean, it's essential to be able to play with them, to rearrange them at will. So in this situation too, to decode the information contained in patterns like this, watching alone won't do. We need to rearrange the pattern. In other words, instead of recording the activity of neurons, we need to control it. It's not essential that we can control the activity of all neurons in the brain, just some. The more targeted our interventions, the better. And I'll show you in a moment how we can achieve the necessary precision.
And since I'm realistic, rather than grandiose, I don't claim that the ability to control the function of the nervous system will at once unravel all its mysteries. But we'll certainly learn a lot. Now, I'm by no means the first person to realize how powerful a tool intervention is. The history of attempts to tinker with the function of the nervous system is long and illustrious. It dates back at least 200 years, to Galvani's famous experiments in the late 18th century and beyond. Galvani showed that a frog's legs twitched when he connected the lumbar nerve to a source of electrical current. This experiment revealed the first, and perhaps most fundamental, nugget of the neural code: that information is written in the form of electrical impulses. Galvani's approach of probing the nervous system with electrodes has remained state-of-the-art until today, despite a number of drawbacks. Sticking wires into the brain is obviously rather crude. It's hard to do in animals that run around, and there is a physical limit to the number of wires that can be inserted simultaneously.
So around the turn of the last century, I started to think, "Wouldn't it be wonderful if one could take this logic and turn it upside down?" So instead of inserting a wire into one spot of the brain, re-engineer the brain itself so that some of its neural elements become responsive to diffusely broadcast signals such as a flash of light. Such an approach would literally, in a flash of light, overcome many of the obstacles to discovery. First, it's clearly a non-invasive, wireless form of communication. And second, just as in a radio broadcast, you can communicate with many receivers at once. You don't need to know where these receivers are, and it doesn't matter if these receivers move -- just think of the stereo in your car. It gets even better, for it turns out that we can fabricate the receivers out of materials that are encoded in DNA. So each nerve cell with the right genetic makeup will spontaneously produce a receiver that allows us to control its function. I hope you'll appreciate the beautiful simplicity of this concept. There's no high-tech gizmos here, just biology revealed through biology.
Now let's take a look at these miraculous receivers up close. As we zoom in on one of these purple neurons, we see that its outer membrane is studded with microscopic pores. Pores like these conduct electrical current and are responsible for all the communication in the nervous system. But these pores here are special. They are coupled to light receptors similar to the ones in your eyes. Whenever a flash of light hits the receptor, the pore opens, an electrical current is switched on, and the neuron fires electrical impulses. Because the light-activated pore is encoded in DNA, we can achieve incredible precision. This is because, although each cell in our bodies contains the same set of genes, different mixes of genes get turned on and off in different cells. You can exploit this to make sure that only some neurons contain our light-activated pore and others don't. So in this cartoon, the bluish white cell in the upper-left corner does not respond to light because it lacks the light-activated pore. The approach works so well that we can write purely artificial messages directly to the brain. In this example, each electrical impulse, each deflection on the trace, is caused by a brief pulse of light. And the approach, of course, also works in moving, behaving animals.
This is the first ever such experiment, sort of the optical equivalent of Galvani's. It was done six or seven years ago by my then graduate student, Susana Lima. Susana had engineered the fruit fly on the left so that just two out of the 200,000 cells in its brain expressed the light-activated pore. You're familiar with these cells because they are the ones that frustrate you when you try to swat the fly. They trained the escape reflex that makes the fly jump into the air and fly away whenever you move your hand in position. And you can see here that the flash of light has exactly the same effect. The animal jumps, it spreads its wings, it vibrates them, but it can't actually take off because the fly is sandwiched between two glass plates. Now to make sure that this was no reaction of the fly to a flash it could see, Susana did a simple but brutally effective experiment. She cut the heads off of her flies. These headless bodies can live for about a day, but they don't do much. They just stand around and groom excessively. So it seems that the only trait that survives decapitation is vanity. (Laughter) Anyway, as you'll see in a moment, Susana was able to turn on the flight motor of what's the equivalent of the spinal cord of these flies and get some of the headless bodies to actually take off and fly away. They didn't get very far, obviously. Since we took these first steps, the field of optogenetics has exploded. And there are now hundreds of labs using these approaches.
And we've come a long way since Galvani's and Susana's first successes in making animals twitch or jump. We can now actually interfere with their psychology in rather profound ways, as I'll show you in my last example, which is directed at a familiar question. Life is a string of choices creating a constant pressure to decide what to do next. We cope with this pressure by having brains, and within our brains, decision-making centers that I've called here the "Actor." The Actor implements a policy that takes into account the state of the environment and the context in which we operate. Our actions change the environment, or context, and these changes are then fed back into the decision loop.
Now to put some neurobiological meat on this abstract model, we constructed a simple one-dimensional world for our favorite subject, fruit flies. Each chamber in these two vertical stacks contains one fly. The left and the right halves of the chamber are filled with two different odors, and a security camera watches as the flies pace up and down between them. Here's some such CCTV footage. Whenever a fly reaches the midpoint of the chamber where the two odor streams meet, it has to make a decision. It has to decide whether to turn around and stay in the same odor, or whether to cross the midline and try something new. These decisions are clearly a reflection of the Actor's policy. Now for an intelligent being like our fly, this policy is not written in stone but rather changes as the animal learns from experience. We can incorporate such an element of adaptive intelligence into our model by assuming that the fly's brain contains not only an Actor, but a different group of cells, a "Critic," that provides a running commentary on the Actor's choices. You can think of this nagging inner voice as sort of the brain's equivalent of the Catholic Church, if you're an Austrian like me, or the super-ego, if you're Freudian, or your mother, if you're Jewish.
(Laughter)
Now obviously, the Critic is a key ingredient in what makes us intelligent. So we set out to identify the cells in the fly's brain that played the role of the Critic. And the logic of our experiment was simple. We thought if we could use our optical remote control to activate the cells of the Critic, we should be able, artificially, to nag the Actor into changing its policy. In other words, the fly should learn from mistakes that it thought it had made but, in reality, it had not made. So we bred flies whose brains were more or less randomly peppered with cells that were light addressable. And then we took these flies and allowed them to make choices. And whenever they made one of the two choices, chose one odor, in this case the blue one over the orange one, we switched on the lights. If the Critic was among the optically activated cells, the result of this intervention should be a change in policy. The fly should learn to avoid the optically reinforced odor.
Here's what happened in two instances: We're comparing two strains of flies, each of them having about 100 light-addressable cells in their brains, shown here in green on the left and on the right. What's common among these groups of cells is that they all produce the neurotransmitter dopamine. But the identities of the individual dopamine-producing neurons are clearly largely different on the left and on the right. Optically activating these hundred or so cells into two strains of flies has dramatically different consequences. If you look first at the behavior of the fly on the right, you can see that whenever it reaches the midpoint of the chamber where the two odors meet, it marches straight through, as it did before. Its behavior is completely unchanged. But the behavior of the fly on the left is very different. Whenever it comes up to the midpoint, it pauses, it carefully scans the odor interface as if it was sniffing out its environment, and then it turns around. This means that the policy that the Actor implements now includes an instruction to avoid the odor that's in the right half of the chamber. This means that the Critic must have spoken in that animal, and that the Critic must be contained among the dopamine-producing neurons on the left, but not among the dopamine producing neurons on the right.
Through many such experiments, we were able to narrow down the identity of the Critic to just 12 cells. These 12 cells, as shown here in green, send the output to a brain structure called the "mushroom body," which is shown here in gray. We know from our formal model that the brain structure at the receiving end of the Critic's commentary is the Actor. So this anatomy suggests that the mushroom bodies have something to do with action choice. Based on everything we know about the mushroom bodies, this makes perfect sense. In fact, it makes so much sense that we can construct an electronic toy circuit that simulates the behavior of the fly. In this electronic toy circuit, the mushroom body neurons are symbolized by the vertical bank of blue LEDs in the center of the board. These LED's are wired to sensors that detect the presence of odorous molecules in the air. Each odor activates a different combination of sensors, which in turn activates a different odor detector in the mushroom body. So the pilot in the cockpit of the fly, the Actor, can tell which odor is present simply by looking at which of the blue LEDs lights up.
What the Actor does with this information depends on its policy, which is stored in the strengths of the connection, between the odor detectors and the motors that power the fly's evasive actions. If the connection is weak, the motors will stay off and the fly will continue straight on its course. If the connection is strong, the motors will turn on and the fly will initiate a turn. Now consider a situation in which the motors stay off, the fly continues on its path and it suffers some painful consequence such as getting zapped. In a situation like this, we would expect the Critic to speak up and to tell the Actor to change its policy. We have created such a situation, artificially, by turning on the critic with a flash of light. That caused a strengthening of the connections between the currently active odor detector and the motors. So the next time the fly finds itself facing the same odor again, the connection is strong enough to turn on the motors and to trigger an evasive maneuver.
I don't know about you, but I find it exhilarating to see how vague psychological notions evaporate and give rise to a physical, mechanistic understanding of the mind, even if it's the mind of the fly. This is one piece of good news. The other piece of good news, for a scientist at least, is that much remains to be discovered. In the experiments I told you about, we have lifted the identity of the Critic, but we still have no idea how the Critic does its job. Come to think of it, knowing when you're wrong without a teacher, or your mother, telling you, is a very hard problem. There are some ideas in computer science and in artificial intelligence as to how this might be done, but we still haven't solved a single example of how intelligent behavior springs from the physical interactions in living matter. I think we'll get there in the not too distant future.
Thank you.
(Applause)
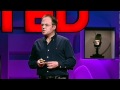
ここに私のドッペルゲンガーがいます。
(笑い) ドクター・ゲロは素晴らしくも 少し狂気じみた科学者です。 ドラゴンボールZの人造人間編に登場します。 よく見てみると、 彼の頭がい骨は アクリルガラスのドームに付け替えられています こうすることで脳の動きが観察できますし、 光でコントロールすることもできます。 まさしくこれが私がやっていること… 光でマインドコントロールすることです。 (笑い)
しかし世界征服を望む この双子の兄弟と違って、 私の野望は邪悪なものではありません。 脳をコントロールすることによって どのように動くかを理解するのです。 ちょっと待てよ、とあなたは言うかもしれません 理解することもしないで、 どのようにコントロールするのか? 客車を馬の前に置くようなものではないか? 多くの神経学者はこの考えに賛成しており、 理解するためには、 詳細な観察と分析が必要と考えている。 彼らは、「もしニューロンの活動を記録できれば、 脳を理解できるだろう」と言っている。 しかし、その意味を少し考えてください。 もし全ての細胞が 何をしているかを常に計測できたとしても、 そのパターンが何であるかを 導き出さなければならない。 それはとても難しく パターンを理解できたとしても、 私たちの脳への理解と同じくらいのものでしょう。
脳の活動がどんなものかご覧に入れましょう。 このシミュレーションでは、一つの黒い点が 一つの神経細胞です。 黒い点は、 細胞が電気信号を発生させるときのみ見えます。 ここには1万個のニューロンがあります。 それは、大体1%くらい ゴキブリの脳の。 皆さんの脳はこれの約1億倍 複雑です。 このようなパターンは、 あなたにも言えることです、 あなたの知覚だったり、 感情、記憶 将来の展望がこれらのパターンなのです。 しかしパターンの解読方法を知らないがために これらが保存されている場所を私たちは知りえません。 脳が使っている暗号を私たちは知りません。 前進するためには、 暗号を解読しなければなりません。 どうやって? 暗号解読のエキスパートは、こう言います 暗号の中のパターンを見つけるためには、 それらを使ってみて、組みなおすことが 大切だ、と。 ここでも同じことが言えます。 このようなパターンに隠されている 情報を解読するためには、 見ているだけではだめで、 組みなおさなければいけない。 別の言葉で言うと ニューロンの活動を記録する代わりに、 制御しなければならない。 必ずしも全ての ニューロンを制御する必要はない。少しでいい。 ターゲットは絞れるだけ絞ったほうがいいです。 今お見せするのは、 どのようにターゲットを絞ったかです。
私は壮大なことより現実的なことを好むので、 神経系統を制御することで瞬く間に 全ての謎が明らかになるとは言いません。 それでも私たちは多くを学ぶことができます。 介入が大きな影響力を 持っていると気づいたのは もちろん私が最初ではありません。 神経系の機能に対する さまざまな試みは 古くから有名です。 少なくとも200年さかのぼって、 ガルバーニの有名な実験は 18世紀以前の話です。 ガルバーニは蛙の腰神経を 電極につなげることで 脚がケイレンすることを示したのです。 この実験は最初でもっとも基本的な 神経コードの大事な部分、 情報は電気信号によって伝えられている ということを明らかにしました。 ガルバーニのとった方法、 電極で神経系を明らかにしようというのは 今日でも先端をいっています、 様々な欠点があるにもかかわらず。 ワイヤを脳に挿すのはどう見ても残酷です。 動き回る動物にやるのは困難ですし、 同時に挿せる ワイヤの数も 限られてきます。
20世紀も終わりのころ、 私はこう考えました。 もしこのロジックを逆に してみたらどうだろう、と。 脳の一部にワイヤを 挿す代わりに、 脳自体を作ってしまおう、 ある数の神経細胞を フラッシュのような拡散する刺激を受けたときに 反応するように。 この試みはまさしくフラッシュのように 様々な障害を取り払ってくれました。 一つ目は、ワイヤがないために 脳を侵さないこと。 二つ目は、ラジオのブロードキャスト放送のように 一度に多くのものとコミュニケーションができること。 どこに受信者がいるか分からなくても大丈夫です。 そして、受信者が動いても大丈夫、ちょうど 車のステレオと同じように。 さらなる長所として、 DNAに組み込まれているものから受信機を 作れることが明らかになりました。 つまり、DNAで正しく 構成されている神経細胞であれば、 受信機を自発的に生成し、 私たちが制御することができます。 このシンプルさに秘められた 美しさを理解していただけると 幸いです。 ハイテクな機械は何もありません、 生態が生物学で明らかになっただけです。
それでは、この奇跡的な受信機を間近で見てみましょう。 これらの紫色のニューロンに近づいていくと、 細胞膜が微小な穴で 覆われているのが見えます。 このような穴が電流を伝え、 神経系統の 全てのコミュニケーションの鍵となっています。 しかし、これらの穴は特別なものです。 これらはあなたの目と同じように、 光の受容体と対になっていて、 光が受容体に当たると 穴が開き電流が伝えられ、 ニューロンが電気信号を発信します。 光で活性化するニューロンはDNAに組み込まれているため、 奇跡的な精度を導き出せます。 なぜなら、 私たちの体の各細胞は 同じ遺伝情報を持っていますが、 細胞によって活性化している箇所が違うために 起こります。 これを利用して、あるニューロンだけを 光で活性化するようにして、 他のものはしないようにできます。 この漫画では、左上の青みがかった 白い細胞は 光に反応しません。 なぜなら光で活性化する穴が欠如しているからです。 この試みは成功し、 直接脳に人工的な文字を 書くこともできます。 この例では、それぞれの電気信号や その跡の偏差は ちょっとした光が引き起こしています。 この方法は、動いたり 行動している動物にも効果的です。
これは、ある意味ガルバーニの実験を 光(レーザー)で行った最初の実験といえます。 これは6、7年前に私のところの 大学院生であったスザーナ・リナによって行われました。 スザーナは左側のミバエの脳を 20万個の細胞のうち2個だけが光に反応するように 遺伝子操作で作りかえました。 これらがあの馴染み深い 叩こうとするときにあなたをイライラさせる 原因となっている細胞達です。 これらがハエの反射運動を引き起こし、私たちが身構えたときには 飛びさってしまうように仕向けているのです。 この実験では、フラッシュが同じ現象を引き起こしています。 飛び跳ねて羽を広げ、それをふるわせていますが、 ガラスの板に挟まれているために 実際には飛び立てません。 ハエが光を見ているためにこの現象が起こっているわけではないと 証明するために、 スザーナはシンプルですが とても有効な実験手段をとりました。 ハエの頭を落としてしまうのです。 頭がない状態でもおよそ1日は生きることができます。 大したことはできませんが。 ただ動き回って、 過剰に身繕いをします。 首が落ちた後に残ったのは"見え"だけのようです。 (笑い) いずれにせよお見せするとおり、 スザーナはハエの脊髄神経から 飛行を司っている部分を制御することに成功し、 頭がないハエを実際に 飛ばしてみせたのです。 遠くに行けないのは当然ですが。 最初の一歩を私たちが始めたことにより、 光遺伝子学の分野が急成長しました。 今では数百の研究所が この実験方法を採用しています。
ガルバーニやスザーナが 動物のケイレンや飛行などの最初の成功を収めてから 長い道を歩んできました。 彼らの心理学に対して少し難解な方法で アプローチしてみましょう。 この最後の例でお見せするのは よく耳にする質問である 人生とは次になすべきことを 常に決め続けさせられた選択の積み重ねである、というものからです。 私たちはこういったプレッシャーに対して脳を使って対処します。 この脳の中で決定の鍵を握るところを 私はアクターと呼んでいます。 アクターはその時に私たちが置かれている状況や 環境を基にした 行動を可能にします。 自分の行動が環境や状況を変えて、 さらにそれらがフィードバックされ、行動が決定されるというループができます。
この神経生物学の議論を 抽象的なモデルに当てはめてみましょう。 私たちお得意のミバエを使い、 一次元の世界を作ってみました。 この垂直に立っている二列のケースそれぞれには 一匹ずつハエが入っています。 それぞれの部屋の右半分と左半分は それぞれ別の匂いで満たされています。 そしてハエが行ったり来たりするのを 監視カメラが見ています。 これが監視カメラがとらえた映像です。 ハエは部屋の真ん中に来たとき、 違う匂いの狭間で 決断をしなければなりません。 向きを変えて今来た道を戻り 同じ匂いに留まるか、 真ん中を越えて 新しいものに挑むか。 この決定はアクターが作り出した 行動方針に従います。 このハエのような知的生物の行動方針は 明文化されているものではなく、 経験から学習し変化していくものです。 この適応性を私たちのモデルに 取り入れるときには アクターとは異なるグループの細胞、 「クリティック」というアクターがした 選択に対してフィードバックをする ものが脳内にあると 仮定しています。 あなたは途絶えることのないこの内なる声、 まるで脳内の教会を 思い浮かべることができるでしょう、もし あなたが私と同じオーストリア人か、 フロイト派の超自我、もしくは ユダヤ人であればお母さんを想像してください。
(笑い)
クリティックが 私たちの知性の鍵と なっていることは明らかです。 そこで私たちはハエの脳で クリティックの役目を果たしている 細胞を特定しようとしました。 実験方法は単純です。 光でクリティックの細胞を遠隔制御して 活性化することができれば、 人為的にアクターの行動方針を変えることが できるはずと考えたのです。 言い換えると、 ハエは自分がした間違いから学習 しているはずですが、 実際には経験していないのです。 私たちは 光で制御できる細胞を無規則で脳にちりばめた ハエを育てました。 これらのハエを使い、 選択をさせました。 2つの選択肢から1つ、 どちらかの匂いを選ぶと、 このケースではオレンジ色ではなく青色の方です、 光をつけます、 光で活性化する細胞の中にクリティックが含まれていれば、 こん介入行動によって 行動方針が変わるはずです。 ハエは光で刺激を受けた方の 匂いを避けるはずです。
例を2つ紹介します。 2系統のハエを比べています、 いずれも 光で制御できる細胞がおよそ100個脳内にあります。 右と左に緑色に見えるものです。 これらのグループに共通しているのは、 いずれも神経伝達物質ドーパミンを生成することです。 しかしドーパミンを生成する ニューロンの性質は 右と左で明らかに大きく異なります。 光でこれら100個かそこらの 細胞を活性化することで 二種類のハエの間には 劇的な違いがうまれます。 右側のハエの行動を 最初に見ると、 ハエが部屋の真ん中、二つの匂いが混じっているところに たどり着くと、 今までと同じようにまっすぐ進みます。 行動は全く変わりません。 しかし、左側のハエは全く違う行動をとります。 真ん中までくると 立ち止まり、 あたかも周りの環境を探り出すかのように 綿密に匂いを分析し、 そして振り返ります。 これらの結果はアクターが作った行動方針が 部屋の右半分に満たされている匂いを 避けるように仕向けているからです。 左側のハエではクリティックが 機能しており、 ドーパミンが生成したニューロンに クリティックが含まれているはずですが、 右側のハエのドーパミンが生成したニューロンには含まれていないということです。
幾多のこのような実験を繰り返し、 クリティックの所在を 12個までに 絞り込みました。 これらの12個の細胞、緑色のところは、 出力情報を脳機構の キノコ体と呼ばれるところ、 この灰色のところ、に送ります。 理論的なモデルから 脳機構の中で クリティックの出力を受けるのはアクターと分かっているので、 この分析が示すところは キノコ体は行動の選択に何かしら 関係があるということです。 キノコ体について分かっていることを考えると 完全に筋が通ります。 実際、あまりにも綺麗に筋が通っているので、 電気回路でハエの行動を模倣する おもちゃを作ることができます。 この電気回路のおもちゃは キノコ体のニューロンは 板の真ん中に垂直な青色LEDの かたまりで表されています。 これらのLEDはセンサーにつながっており 空気中の匂い分子の存在を感知します。 それぞれの匂いはそれぞれ違うパターンのセンサーを活性化させ それはキノコ体の中の それぞれ異なる匂い検知器官を活性化させます。 ハエの操縦席ではアクターという パイロットが どの匂いがあるのかを どの青色LEDが光っているかを見ることで認識することができます。
アクターが個の情報を基に何をするかは 行動方針によって変わり、 匂い検知器官とハエの回避行動を起こしている 伝達細胞の結びつきの強さが その方針の より所になっています。 もし結びつきが弱ければ伝達細胞は動かず、 ハエはそのまま直進します。 結びつきが強ければ伝達細胞が動き、 ハエは今来た道を戻ります。 次のような状況を考えてみましょう。 伝達細胞が動かないまま まっすぐ進むと、 痛みを伴う結果が待っている、 例えば叩かれるような。 このような状況では、 クリティックは行動方針を変えるよう アクターに働きかけると 予想しました。 この状況をフラッシュをたくことで人為的に 作り出しました。 そしてこれは現在活性化している匂い検知器官と 伝達細胞の結びつきを強める 結果となりました。 つまり次に ハエが同じ匂いと出くわしたとき、 結びつきは伝達器官を動かし、 回避行動をとります。
私はあなたのことを知りませんが、 心理学の抽象的な 概念が消え失せ、 精神を実態的、 機能的に理解することがとても楽しいことを発見しました。 たとえハエの精神だとしても。 これはよいニュースの一つです。 もう一つのよいニュースは、 少なくとも科学者にとっては、 まだまだ未知の領域があるということです。 前述した実験で、 クリティックの所在はつかめましたが、 クリティックがどのように 動いているかはまだ分かりません。 考えてみると、先生やお母さんの助言無しでは 自分の間違いに気づくことは とても難しいことです。 コンピュータサイエンスや人工知能の 中にはこれらの解決策の 糸口がありますが、 生物同士の 接触から生じる 知的行動に関しては、 何一つ証明が できていません。 それらが解決されるのもそう遠くないと思っています。
ありがとう
(拍手)
品詞分類
- 主語
- 動詞
- 助動詞
- 準動詞
- 関係詞等
TED 日本語
TED Talks
関連動画
うつやPTSDを予防できる新種の薬 | TED Talkレベッカ・ブラックマン
2019.04.17騒音が健康に有害な理由 ― そして私たちにできることマティアス・バスナー
2019.02.26さまよう心を鎮めるには | TED Talkアミシ・ジャー
2018.04.18鬱の友達と心を通わせるにはビル・バーナット
2018.03.23脳に良い変化をもたらす運動の効果ウェンディー・スズキ
おすすめ 12018.03.21物事の「良し悪し」は思い込みに過ぎないヘザー・ラニエ
おすすめ 12018.01.19脳が深い睡眠から更なる恩恵を得る方法ダン・ガーテンバーグ
2018.01.04うつを一人で抱え込まないでニッキー・ウェバー・アレン
2017.10.26長寿の秘訣は周囲の人との交流かもスーザン・ピンカー
2017.09.04脳はどのように美しさを判定するか?アンジャン・チャタジー
2017.08.22脳が「意識された現実」という幻覚を作り出す仕組みアニル・セス
2017.07.18注意を向けた時、脳では何が起きているのかメディ・オディカニ=セイドラー
2017.07.12ティーンエイジャーの登校時間を遅らせるべき理由とはウェンディ・トロクセル
2017.06.09死に直面したとき、人生に生きる価値を与えてくれるのはルーシー・カラニシ
おすすめ 12017.06.07最善の自己と最悪の自己の生物学ロバート・サポルスキー
2017.05.31メンタルヘルスを気遣うのは恥ずかしくなんかないサング・デリ
2017.05.26
洋楽 おすすめ
RECOMMENDS
洋楽歌詞
ダイナマイトビーティーエス
洋楽最新ヒット2020.08.20ディス・イズ・ミーグレイテスト・ショーマン・キャスト
洋楽人気動画2018.01.11グッド・ライフGイージー、ケラーニ
洋楽人気動画2017.01.27ホワット・ドゥ・ユー・ミーン?ジャスティン・ビーバー
洋楽人気動画2015.08.28ファイト・ソングレイチェル・プラッテン
洋楽人気動画2015.05.19ラヴ・ミー・ライク・ユー・ドゥエリー・ゴールディング
洋楽人気動画2015.01.22アップタウン・ファンクブルーノ・マーズ、マーク・ロンソン
洋楽人気動画2014.11.20ブレイク・フリーアリアナ・グランデ
洋楽人気動画2014.08.12ハッピーファレル・ウィリアムス
ポップス2014.01.08カウンティング・スターズワンリパブリック
ロック2013.05.31ア・サウザンド・イヤーズクリスティーナ・ペリー
洋楽人気動画2011.10.26ユー・レイズ・ミー・アップケルティック・ウーマン
洋楽人気動画2008.05.30ルーズ・ユアセルフエミネム
洋楽人気動画2008.02.21ドント・ノー・ホワイノラ・ジョーンズ
洋楽人気動画2008.02.15オンリー・タイムエンヤ
洋楽人気動画2007.10.03ミス・ア・シングエアロスミス
ロック2007.08.18タイム・トゥ・セイ・グッバイサラ・ブライトマン
洋楽人気動画2007.06.08シェイプ・オブ・マイ・ハートスティング
洋楽人気動画2007.03.18ウィ・アー・ザ・ワールド(U.S.A. フォー・アフリカ)マイケル・ジャクソン
洋楽人気動画2006.05.14ホテル・カリフォルニアイーグルス
ロック2005.07.06