
TED日本語
TED Talks(英語 日本語字幕付き動画)
TED日本語 - エド・ボイデン: ニューロンの光スイッチ
TED Talks
ニューロンの光スイッチ
A light switch for neurons
エド・ボイデン
Ed Boyden
内容
感光性タンパク質の遺伝子を脳細胞に導入し、移植したファイバー光学系を用いて特定のニューロンを選択的にオンオフできることをエド・ボイデンが示します。この先例のない制御技術によって、PTSD やある種の失明のマウスモデルを治療することにも成功しました。神経補装具という新たな地平線が見えてきました。セッション司会のファン・エンリケズによる簡単な質疑応答も収録。
字幕
SCRIPT
Script
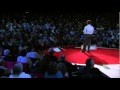
Think about your day for a second. You woke up, felt fresh air on your face as you walked out the door, encountered new colleagues and had great discussions, and felt in awe when you found something new. But I bet there's something you didn't think about today -- something so close to home that you probably don't think about it very often at all. And that's that all the sensations, feelings, decisions and actions are mediated by the computer in your head called the brain.
Now the brain may not look like much from the outside -- a couple pounds of pinkish-gray flesh, amorphous -- but the last hundred years of neuroscience have allowed us to zoom in on the brain, and to see the intricacy of what lies within. And they've told us that this brain is an incredibly complicated circuit made out of hundreds of billions of cells called neurons. Now unlike a human-designed computer, where there's a fairly small number of different parts -- we know how they work, because we humans designed them -- the brain is made out of thousands of different kinds of cells, maybe tens of thousands. They come in different shapes; they're made out of different molecules. And they project and connect to different brain regions, and they also change different ways in different disease states.
Let's make it concrete. There's a class of cells, a fairly small cell, an inhibitory cell, that quiets its neighbors. It's one of the cells that seems to be atrophied in disorders like schizophrenia. It's called the basket cell. And this cell is one of the thousands of kinds of cell that we are learning about. New ones are being discovered everyday. As just a second example: these pyramidal cells, large cells, they can span a significant fraction of the brain. They're excitatory. And these are some of the cells that might be overactive in disorders such as epilepsy. Every one of these cells is an incredible electrical device. They receive input from thousands of upstream partners and compute their own electrical outputs, which then, if they pass a certain threshold, will go to thousands of downstream partners. And this process, which takes just a millisecond or so, happens thousands of times a minute in every one of your 100 billion cells, as long as you live and think and feel.
So how are we going to figure out what this circuit does? Ideally, we could go through the circuit and turn these different kinds of cell on and off and see whether we could figure out which ones contribute to certain functions and which ones go wrong in certain pathologies. If we could activate cells, we could see what powers they can unleash, what they can initiate and sustain. If we could turn them off, then we could try and figure out what they're necessary for. And that's a story I'm going to tell you about today. And honestly, where we've gone through over the last 11 years, through an attempt to find ways of turning circuits and cells and parts and pathways of the brain on and off, both to understand the science and also to confront some of the issues that face us all as humans.
Now before I tell you about the technology, the bad news is that a significant fraction of us in this room, if we live long enough, will encounter, perhaps, a brain disorder. Already, a billion people have had some kind of brain disorder that incapacitates them, and the numbers don't do it justice though. These disorders -- schizophrenia, Alzheimer's, depression, addiction -- they not only steal our time to live, they change who we are. They take our identity and change our emotions and change who we are as people. Now in the 20th century, there was some hope that was generated through the development of pharmaceuticals for treating brain disorders, and while many drugs have been developed that can alleviate symptoms of brain disorders, practically none of them can be considered to be cured. And part of that's because we're bathing the brain in the chemical. This elaborate circuit made out of thousands of different kinds of cell is being bathed in a substance. That's also why, perhaps, most of the drugs, and not all, on the market can present some kind of serious side effect too.
Now some people have gotten some solace from electrical stimulators that are implanted in the brain. And for Parkinson's disease, Cochlear implants, these have indeed been able to bring some kind of remedy to people with certain kinds of disorder. But electricity also will go in all directions -- the path of least resistance, which is where that phrase, in part, comes from. And it also will affect normal circuits as well as the abnormal ones that you want to fix. So again, we're sent back to the idea of ultra-precise control. Could we dial-in information precisely where we want it to go?
So when I started in neuroscience 11 years ago, I had trained as an electrical engineer and a physicist, and the first thing I thought about was, if these neurons are electrical devices, all we need to do is to find some way of driving those electrical changes at a distance. If we could turn on the electricity in one cell, but not its neighbors, that would give us the tool we need to activate and shut down these different cells, figure out what they do and how they contribute to the networks in which they're embedded. And also it would allow us to have the ultra-precise control we need in order to fix the circuit computations that have gone awry. Now how are we going to do that? Well there are many molecules that exist in nature, which are able to convert light into electricity. You can think of them as little proteins that are like solar cells. If we can install these molecules in neurons somehow, then these neurons would become electrically drivable with light. And their neighbors, which don't have the molecule, would not. There's one other magic trick you need to make this all happen, and that's the ability to get light into the brain. And to do that -- the brain doesn't feel pain -- you can put -- taking advantage of all the effort that's gone into the Internet and communications and so on -- optical fibers connected to lasers that you can use to activate, in animal models for example, in pre-clinical studies, these neurons and to see what they do.
So how do we do this? Around 2004, in collaboration with Gerhard Nagel and Karl Deisseroth, this vision came to fruition. There's a certain alga that swims in the wild, and it needs to navigate towards light in order to photosynthesize optimally. And it senses light with a little eye-spot, which works not unlike how our eye works. In its membrane, or its boundary, it contains little proteins that indeed can convert light into electricity. So these molecules are called channelrhodopsins. And each of these proteins acts just like that solar cell that I told you about. When blue light hits it, it opens up a little hole and allows charged particles to enter the eye-spot, and that allows this eye-spot to have an electrical signal just like a solar cell charging up a battery.
So what we need to do is to take these molecules and somehow install them in neurons. And because it's a protein, it's encoded for in the DNA of this organism. So all we've got to do is take that DNA, put it into a gene therapy vector, like a virus, and put it into neurons. So it turned out that this was a very productive time in gene therapy, and lots of viruses were coming along. So this turned out to be very simple to do. And early in the morning one day in the summer of 2004, we gave it a try, and it worked on the first try. You take this DNA and you put it into a neuron. The neuron uses its natural protein-making machinery to fabricate these little light-sensitive proteins and install them all over the cell, like putting solar panels on a roof, and the next thing you know, you have a neuron which can be activated with light. So this is very powerful.
One of the tricks you have to do is to figure out how to deliver these genes to the cells that you want and not all the other neighbors. And you can do that; you can tweak the viruses so they hit just some cells and not others. And there's other genetic tricks you can play in order to get light-activated cells. This field has now come to be known as optogenetics. And just as one example of the kind of thing you can do, you can take a complex network, use one of these viruses to deliver the gene just to one kind of cell in this dense network. And then when you shine light on the entire network, just that cell type will be activated.
So for example, lets sort of consider that basket cell I told you about earlier -- the one that's atrophied in schizophrenia and the one that is inhibitory. If we can deliver that gene to these cells -- and they're not going to be altered by the expression of the gene, of course -- and then flash blue light over the entire brain network, just these cells are going to be driven. And when the light turns off, these cells go back to normal, so they don't seem to be averse against that. Not only can you use this to study what these cells do, what their power is in computing in the brain, but you can also use this to try to figure out -- well maybe we could jazz up the activity of these cells, if indeed they're atrophied.
Now I want to tell you a couple of short stories about how we're using this, both at the scientific, clinical and pre-clinical levels. One of the questions we've confronted is, what are the signals in the brain that mediate the sensation of reward? Because if you could find those, those would be some of the signals that could drive learning. The brain will do more of whatever got that reward. And also these are signals that go awry in disorders such as addiction. So if we could figure out what cells they are, we could maybe find new targets for which drugs could be designed or screened against, or maybe places where electrodes could be put in for people who have very severe disability. So to do that, we came up with a very simple paradigm in collaboration with the Fiorella group, where one side of this little box, if the animal goes there, the animal gets a pulse of light in order to make different cells in the brain sensitive to light. So if these cells can mediate reward, the animal should go there more and more. And so that's what happens.
This animal's going to go to the right-hand side and poke his nose there, and he gets a flash of blue light every time he does that. And he'll do that hundreds and hundreds of times. These are the dopamine neurons, which some of you may have heard about, in some of the pleasure centers in the brain. Now we've shown that a brief activation of these is enough, indeed, to drive learning. Now we can generalize the idea. Instead of one point in the brain, we can devise devices that span the brain, that can deliver light into three-dimensional patterns -- arrays of optical fibers, each coupled to its own independent miniature light source. And then we can try to do things in vivo that have only been done to-date in a dish -- like high-throughput screening throughout the entire brain for the signals that can cause certain things to happen. Or that could be good clinical targets for treating brain disorders.
And one story I want to tell you about is how can we find targets for treating post-traumatic stress disorder -- a form of uncontrolled anxiety and fear. And one of the things that we did was to adopt a very classical model of fear. This goes back to the Pavlovian days. It's called Pavlovian fear conditioning -- where a tone ends with a brief shock. The shock isn't painful, but it's a little annoying. And over time -- in this case, a mouse, which is a good animal model, commonly used in such experiments -- the animal learns to fear the tone. The animal will react by freezing, sort of like a deer in the headlights. Now the question is, what targets in the brain can we find that allow us to overcome this fear? So what we do is we play that tone again after it's been associated with fear. But we activate targets in the brain, different ones, using that optical fiber array I told you about in the previous slide, in order to try and figure out which targets can cause the brain to overcome that memory of fear.
And so this brief video shows you one of these targets that we're working on now. This is an area in the prefrontal cortex, a region where we can use cognition to try to overcome aversive emotional states. And the animal's going to hear a tone -- and a flash of light occurred there. There's no audio on this, but you can see the animal's freezing. This tone used to mean bad news. And there's a little clock in the lower left-hand corner, so you can see the animal is about two minutes into this. And now this next clip is just eight minutes later. And the same tone is going to play, and the light is going to flash again. Okay, there it goes. Right now. And now you can see, just 10 minutes into the experiment, that we've equipped the brain by photoactivating this area to overcome the expression of this fear memory.
Now over the last couple of years, we've gone back to the tree of life because we wanted to find ways to turn circuits in the brain off. If we could do that, this could be extremely powerful. If you can delete cells just for a few milliseconds or seconds, you can figure out what necessary role they play in the circuits in which they're embedded. And we've now surveyed organisms from all over the tree of life -- every kingdom of life except for animals, we see slightly differently. And we found all sorts of molecules, they're called halorhodopsins or archaerhodopsins, that respond to green and yellow light. And they do the opposite thing of the molecule I told you about before with the blue light activator channelrhodopsin.
Let's give an example of where we think this is going to go. Consider, for example, a condition like epilepsy, where the brain is overactive. Now if drugs fail in epileptic treatment,one of the strategies is to remove part of the brain. But that's obviously irreversible, and there could be side effects. What if we could just turn off that brain for a brief amount of time, until the seizure dies away, and cause the brain to be restored to its initial state -- sort of like a dynamical system that's being coaxed down into a stable state. So this animation just tries to explain this concept where we made these cells sensitive to being turned off with light, and we beam light in, and just for the time it takes to shut down a seizure, we're hoping to be able to turn it off. And so we don't have data to show you on this front, but we're very excited about this.
Now I want to close on one story, which we think is another possibility -- which is that maybe these molecules, if you can do ultra-precise control, can be used in the brain itself to make a new kind of prosthetic, an optical prosthetic. I already told you that electrical stimulators are not uncommon. Seventy-five thousand people have Parkinson's deep-brain stimulators implanted. Maybe 100,000 people have Cochlear implants, which allow them to hear. There's another thing, which is you've got to get these genes into cells. And new hope in gene therapy has been developed because viruses like the adeno-associated virus, which probably most of us around this room have, and it doesn't have any symptoms, which have been used in hundreds of patients to deliver genes into the brain or the body. And so far, there have not been serious adverse events associated with the virus.
There's one last elephant in the room, the proteins themselves, which come from algae and bacteria and fungi, and all over the tree of life. Most of us don't have fungi or algae in our brains, so what is our brain going to do if we put that in? Are the cells going to tolerate it? Will the immune system react? In its early days -- these have not been done on humans yet -- but we're working on a variety of studies to try and examine this, and so far we haven't seen overt reactions of any severity to these molecules or to the illumination of the brain with light. So it's early days, to be upfront, but we're excited about it.
I wanted to close with one story, which we think could potentially be a clinical application. Now there are many forms of blindness where the photoreceptors, our light sensors that are in the back of our eye, are gone. And the retina, of course, is a complex structure. Now let's zoom in on it here, so we can see it in more detail. The photoreceptor cells are shown here at the top, and then the signals that are detected by the photoreceptors are transformed by various computations until finally that layer of cells at the bottom, the ganglion cells, relay the information to the brain, where we see that as perception. In many forms of blindness, like retinitis pigmentosa, or macular degeneration, the photoreceptor cells have atrophied or been destroyed. Now how could you repair this? It's not even clear that a drug could cause this to be restored, because there's nothing for the drug to bind to. On the other hand, light can still get into the eye. The eye is still transparent and you can get light in. So what if we could just take these channelrhodopsins and other molecules and install them on some of these other spare cells and convert them into little cameras. And because there's so many of these cells in the eye, potentially, they could be very high-resolution cameras.
So this is some work that we're doing. It's being led by one of our collaborators, Alan Horsager at USC, and being sought to be commercialized by a start-up company Eos Neuroscience, which is funded by the NIH. And what you see here is a mouse trying to solve a maze. It's a six-arm maze. And there's a bit of water in the maze to motivate the mouse to move, or he'll just sit there. And the goal, of course, of this maze is to get out of the water and go to a little platform that's under the lit top port. Now mice are smart, so this mouse solves the maze eventually, but he does a brute-force search. He's swimming down every avenue until he finally gets to the platform. So he's not using vision to do it. These different mice are different mutations that recapitulate different kinds of blindness that affect humans. And so we're being careful in trying to look at these different models so we come up with a generalized approach.
So how are we going to solve this? We're going to do exactly what we outlined in the previous slide. We're going to take these blue light photosensors and install them on a layer of cells in the middle of the retina in the back of the eye and convert them into a camera -- just like installing solar cells all over those neurons to make them light sensitive. Light is converted to electricity on them. So this mouse was blind a couple weeks before this experiment and received one dose of this photosensitive molecule in a virus. And now you can see, the animal can indeed avoid walls and go to this little platform and make cognitive use of its eyes again. And to point out the power of this: these animals are able to get to that platform just as fast as animals that have seen their entire lives. So this pre-clinical study, I think, bodes hope for the kinds of things we're hoping to do in the future.
To close, I want to point out that we're also exploring new business models for this new field of neurotechnology. We're developing these tools, but we share them freely with hundreds of groups all over the world, so people can study and try to treat different disorders. And our hope is that, by figuring out brain circuits at a level of abstraction that lets us repair them and engineer them, we can take some of these intractable disorders that I told you about earlier, practically none of which are cured, and in the 21st century make them history.
Thank you.
(Applause)
Juan Enriquez: So some of the stuff is a little dense. (Laughter) But the implications of being able to control seizures or epilepsy with light instead of drugs, and being able to target those specifically is a first step. The second thing that I think I heard you say is you can now control the brain in two colors, like an on/off switch.
Ed Boyden: That's right.
JE: Which makes every impulse going through the brain a binary code.
EB: Right, yeah. So with blue light, we can drive information, and it's in the form of a one. And by turning things off, it's more or less a zero. So our hope is to eventually build brain coprocessors that work with the brain so we can augment functions in people with disabilities.
JE: And in theory, that means that, as a mouse feels, smells, hears, touches, you can model it out as a string of ones and zeros.
EB: Sure, yeah. We're hoping to use this as a way of testing what neural codes can drive certain behaviors and certain thoughts and certain feelings, and use that to understand more about the brain.
JE: Does that mean that some day you could download memories and maybe upload them?
EB: Well that's something we're starting to work on very hard. We're now working on some work where we're trying to tile the brain with recording elements too. So we can record information and then drive information back in -- sort of computing what the brain needs in order to augment its information processing.
JE: Well, that might change a couple things. Thank you. (EB: Thank you.)
(Applause)
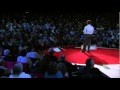
皆さんの日常について考えてみてください 起床し 外に出て清々しい風を感じ 新しい同僚と良い議論をし 新しい発見に感動するでしょう でもたぶん気にも留めなかったことがあるはずです 実に当たり前すぎて 普段はまったく意識されないことです それは感覚や感情 決断や行動などは 頭の中にあって脳と呼ばれるコンピュータが 仕切っているということです
外見上 脳は大したものには見えません 1kg 程度のピンクがかった灰色の 不定形の肉なのですが 過去百年の神経科学の発展により 脳を詳細に観察できるようになり その複雑さを研究できるようになりました その結果 脳は 数千億のニューロンと呼ばれる細胞が織りなす 複雑な回路から成っていることが分かりました 人間が設計したコンピュータの 部品の種類は少ないのですが これは我々が設計したので仕組みは分かっていますが -- 脳は数千種類の多様な細胞から出来ています 数万種類かもしれません 形も違っていますし 構成する分子も違います それぞれが様々な脳部位へと繋がっています また様々な病気で 様々に変化します
具体的にお話ししましょう 近隣細胞を不活性化する 抑制細胞という比較的小さな細胞があります これは統合失調症などで萎縮が見られる細胞です 籠細胞と呼ばれます 我々が研究している数千種類の 細胞の内の一つです 新種の細胞が日々発見されています もう一つ 例として この大きな錐体細胞は 多くの脳部位に存在しています これは興奮性の細胞であり てんかんなどで 過剰活性していると思われる細胞の一つです これらの細胞一つ一つが 驚くべき電気装置なのです 数千個の上流の細胞から入力を受け取り 自身の電気出力を計算し それが一定の閾値を超えている場合 数千個の下流の細胞へ出力します 1ミリ秒ほどで起きるこのプロセスは 1千億個の細胞全てで 毎分何千回も繰り返されます 皆さんが生きていて 考え 感じている限りにおいて
どうしたらこの回路の働きを解明できるでしょう? 理想は 回路を構成している 全細胞をオンオフして どの種類の細胞が どの機能に寄与しているかとか どの病態でおかしくなるか 調べていくことです 細胞を活性化できれば それが何を引き起こし 何を維持するか 調べられます 不活性化できれば それが何に必要な細胞か分かります これが本日 私がお話しする内容です 我々はこれまでの 11 年間 脳の回路 細胞 組織 経路を オンオフする方法を 模索してきました 科学を理解するため また人間として我々が直面する 数々の問題に立ち向かうためにです
技術的なお話をする前に 残念なことに 長生きしていくと 我々はかなりの割合で 脳疾患に罹ります 既に十億人が 機能障害を及ぼす 脳疾患に罹っています この数字だけでは実態を伝えるには不十分です 統合失調症 アルツハイマー病 うつ病 依存症などの障害は 我々の寿命を削るだけでなく 我々自身を変容させます 自己同一性を奪い 感情を変え 人間としての我々を変えます 20 世紀には 脳障害を治療する 薬剤の開発がずいぶん期待されたものでした 脳障害の症状を緩和する たくさんの治療薬が開発される一方 実際に完治できる薬はできませんでした その理由の一つは脳が化学物質に浸かっているためです 数千種類の細胞からなる 脳の精巧な回路は 化学物質に浸っています 全てではないけれど 市販のほとんどの薬が 深刻な副作用を引き起こす理由でしょう
脳に埋め込んだ電気刺激器で ある程度助かっている人もいます パーキンソン病と 人工内耳に関しては この電気刺激機器が ある種の障害に対する 助けとなってきました しかし電流は全方向へ流れます 抵抗の低い部位を 経路として流れていきます 従って電気刺激は治したい異常回路と正常な回路 両方に影響します そうして再び我々は 超精密制御の考えに戻るのです 信号を思い通りに制御できるのか?
11 年前に神経科学を始めたとき 私は電気と物理が専門だったので 最初に考えたことは ニューロンが電気装置ならば その電気的変化を遠隔操作する方法を 見つければよいということでした もし隣接細胞は発火させずに 一つの細胞だけを発火させられたら 様々な細胞を活性および抑制するツールが得られ そして個々が何をし そのネットワーク上で どのように役割を果たしているかが分かります また同時に正常な計算ができなくなった回路を 元通りにするための 超精密制御が可能となります どうしたら実現できるでしょう? 自然界には光を電気へと変換できる分子が 多数存在しています 太陽電池のように働く 小さなタンパク質だと考えてください この分子をどうにかしてニューロンに導入できれば そのニューロンは光刺激で活性化させられます この分子を持たない隣接細胞は反応しません これを実際のものにするには 更に 脳内に光刺激を届けるための工夫が必要です そのために 痛覚のない脳に インターネットや通信技術などの 成果である光ファイバーを挿入します 光ファイバーにニューロンを 活性化させるためのレーザーを接続し 動物を使った前臨床実験で ニューロンの振る舞いを観察します
これはどうやるのでしょうか? 2004 年頃に ゲルハルト・ナゲルとカール・ダイセロスと共同して この構想は実現しました 野性の藻には 適切に光合成を行うために 光へ向かって移動するものが存在します 我々の目とは異なる仕組みの 小さな眼点で光を感知します その細胞膜には 光を電気へと変換できる 小さなタンパク質が含まれています これはチャネルロドプシンと呼ばれるものです このタンパク質は先ほど触れた太陽電池のように振る舞います 青い光で刺激されると小さな穴を開き 荷電粒子を眼点内へ取り込みます それによって太陽電池が充電するのと同様に 眼点は電気信号を溜めます
我々がしなければならなかったのは この分子を抽出しニューロンへ導入することでした それはタンパク質なので その藻の DNA 内にコードされています あとはその DNA を抽出し 遺伝子治療用ベクターというウィルスみたいなものに取り込み それをニューロンに導入すれば良いだけでした これは遺伝子治療が大いに進んだ時期で たくさんのウィルスが登場しており やってみると簡単なことでした 2004 年の夏のある朝に実験し 最初の試行で成功しました この DNA を抽出しニューロンに導入するのです ニューロン自身のタンパク質生成機能が あの感光タンパク質を組み立て ソーラーパネルを設置するが如く 全ての細胞に導入します そうすると 光刺激で活性化させられるニューロンの出来上がりです これは非常に強力です
工夫が必要なのは 隣接細胞ではなく目的の細胞だけに この遺伝子を導入する方法です これは可能です まずウィルスを 特定の細胞にだけ取り付くよう改造します 光で活性化する細胞を作るための 遺伝子の工夫はまだあります この分野は光遺伝学として知られるようになりました その一例として複雑なネットワークを 取り上げてみます 高密度なネットワークに存在する 一種類の細胞にだけ ウィルスを用いて遺伝子を導入できます そしてネットワーク全体に対して光を照らすと その種類の細胞だけが活性化します
例えば先ほどの籠細胞を取り上げてみましょう 統合失調症において萎縮してしまう 抑制系の細胞です この細胞の表現系を変化させずに 先の遺伝子を導入できれば 脳のネットワーク全体に青い光を照射して この細胞だけを活性化できます 照射を止めれば細胞は正常状態に戻るので 特に悪影響もないでしょう この技術を用いれば細胞の働きや 脳全体における役割だけでなく 籠細胞が本当に萎縮しているとしたら そのことを明らかにし 籠細胞の活動を活性化できます
では我々がこの技術を 科学 臨床 前臨床 それぞれの段階で どう利用しているかお話ししたいと思います 我々が取り組んだ問題の一つは 報酬という感覚を脳内で媒介する信号は何かというものです なぜならこれを発見できれば 学習を促進する信号に成り得るからです 脳は報酬を得たことを より実行します またその報酬信号は中毒などの障害では異常になります 従ってどの細胞かを解明できれば 薬の設計やスクリーニングに有効な 新たな創薬ターゲットの発見や 重症患者に対する適切な 電極刺激部位の同定に繋がるかもしれません そのために我々はフィオレッラグループと共同で ある単純なパラダイムを作りました この小さな箱の一方に 動物が移動すると 脳の感光性細胞を活性化する 光のパルスが照射されるようにしました 従って その細胞が報酬を媒介している場合 動物は光が照射される方へ行くようになるはずです そしてその通りの結果が出ました
この動物が右側の穴を鼻で突くと その度に青い光が照射されるようにしました 彼は何百回とこれを繰り返します これらはドーパミンニューロンによるものです 快楽に関わる物質としてご存じの方もいるでしょう 学習を促進するにはこれを少し 活性化すればよいことが分かりました 次に このアイデアを拡張し 脳の一点だけでなく 脳全体に対して立体的に光を照射できる 機器を用意します 独立した小型の光源に接続した 光ファイバーの束を用います これによってシャーレでしか 出来なかったことを生体で実験できます 例えば特定の現象を引き起こす信号の スクリーニングを脳全体に対して実施できます また 脳障害の治療ターゲットの 探索にも利用できます
制御不能な不安や恐怖を示す PTSD の治療ターゲットをどう探すかについて 一つお話ししたいと思います 我々が試したことの一つは 恐怖の古典的モデルを取り入れることでした それはパブロフの時代まで遡ります これはパブロフの条件付けと呼ばれ 音の呈示後に電気ショックをあたえるものでした 痛くはありませんが 少し不快なものでした こういった実験でよく用いられる マウスで繰り返し実験したところ 音を怖がるよう条件付けられました 動物は反射的にこわばります ヘッドライトに照らされたときのシカと同じです ここでの問題は この恐怖を克服するための何かは 脳のどこで見つけられるかということです 何をするかというと 恐怖と条件付けた音を鳴らします ただその時に 先ほどお見せした 光ファイバーを用いて別の脳部位を活性化させ 恐怖の記憶を克服するためには どの脳部位が働くのかを調べます
この簡単なビデオで 我々が取り組んでいるターゲットの一つをお見せします こちらが前頭前野 嫌感情を認知で克服しようとする時に活動する脳部位です 動物は音を聴き そこで光の照射を受けます 音声は入っていませんが動物が硬直しているのは確認できます 音は悪い知らせとして使われます 左下の時計を見ると 実験が始まって2分が過ぎたことが分かります 次のシーンは 8 分後のものです 同じ音刺激が呈示され 光が照射されます 光ります 今です ご覧の通り 10 分の実験で 我々はこの部位を光で 活性化させ 恐怖の記憶を 克服させることが出来ました
我々はこの数年間 生命の樹を振り返っていました 脳内の回路をオフにする方法を探していたのです 実現すれば 極めて有効な手段となります 数ミリ秒あるいは数秒でも細胞を 除けておくことができれば その回路における役割を解明できます 我々は生命の樹全体の生物を調べ 動物以外の界は若干異なることが分かりました また緑と黄色の光に反応するハロロドプシン または古細菌ロドプシンと呼ばれる分子を見つけました これらは先に述べた青い光に反応する チャネルロドプシンとは逆のことをします
これらを上手く利用できる例を挙げます 脳が過剰に活動している てんかんの症状を例に取ってみましょう てんかん治療で薬が有効でなかった場合 他には脳の一部を除去するという手段がありますが それは明らかに不可逆で 副作用も見込まれます もし一時的に発作が止まるまで 脳を停止できたとしたらどうでしょう 力学において動的な系を安定状態に移行させるように 脳を初期状態に戻すのです こちらのアニメーションは その概念の説明です 光刺激によってオフに出来る細胞を作り 光を照射し 発作を鎮めるのにかかる時間だけ 活動を停止させられたらと考えています この最新の研究についてお見せできるデータはありません 張り切って取り組んでいるところです
もう一つの展望として 注目したいのが 超精密制御でこの分子を 脳自体に用いることが出来れば 新たな光学式補装具が出来ると考えています 電気刺激機器が珍しくないことは既にお話ししました 7万5千人のパーキンソン病患者が電気刺激機器を移植しており 補聴器としておそらく 10 万人以上が 人工内耳を移植しています もう一つは遺伝子を細胞に導入する必要があるということです 遺伝子治療における新たな期待として この部屋にいる全員が保有しているであろう 何の症状ももたらさない アデノウィルスが開発され 脳や体に遺伝子を導入するために 百人以上の患者で用いられています これまでこのウィルスによる悪影響は 報告されていません
まだ誰も触れていない大問題として 藻 バクテリア 菌類など 系統樹のあちこちからのタンパク質が問題です 我々は通常脳に菌類や藻類を持っていませんが それを導入したらどうなるでしょう? 許容するでしょうか?排除するでしょうか? まだ人間では実験されていませんが 我々は数々の研究に着手しており これを検証しようとしています まだこれらの分子や 光刺激による脳活性について どのような悪影響も観察されていません 正直 まだ初期段階ですが 我々は興奮しています
最後に 臨床に活用できると思われる お話をして締めたいと思います 我々の目の奥にある 光受容体が無くなってしまう 失明にはいくつもの形態があります そして当然網膜は複雑な構造をしています 拡大してもっと細かく見てみましょう 光受容体細胞は一番上にあり 受け取った信号は細胞の層の 一番下にある神経節に至るまでに 様々な計算を経て変換され そこから脳へ情報が伝達され 我々は映像を知覚します 網膜色素変性や黄斑変性などの 盲目において 光受容体細胞は萎縮または破壊されています どうしたら治せるでしょう? 薬が結合する対象が存在しないため 薬による治療が可能かどうかすら不明です その一方で 光信号は変わらず目に入ってきているのです 光は変わらず素通りしてきています ではもしチャネルロドプシンなどの分子を 代替の細胞などに導入し カメラ代わりに使えたとしたらどうでしょう? 目には多数の細胞が存在するので 解像度の非常に高いカメラとなるはずです
我々はこのようなことをしています これは我々の共同研究者の一人 USC のアラン・ホーセイジャーが率い NIH の資金援助の下 Eos Neuroscience 社の立ち上げと共に 商業化をしようとしています これはマウスが迷路を解こうとしている場面です 6 方向放射状迷路です マウスの活動を促すため 水を少し入れています この迷路の目的は 水から上がって 小さな台へ移動することです マウスは賢いので最終的には迷路を解きますが 総当たりでやります 台にたどり着くまで全ての通路を泳ぎます つまり見通しを立ててはいないのです これらのマウスは遺伝子改変によって 人間の盲目をモデルした変異マウスです それぞれのモデルを慎重に観察し 一般化できる解決手法を模索します
どのようにしたらよいでしょう? 先のスライドで示した通り 我々は青い光センサーを 目の裏の網膜の 細胞層の真ん中に導入し カメラにします 太陽電池を導入し ニューロンを 感光性にしたときと同様です そこで光は電気に変換されます このマウスは実験の数週間前に失明させ 感光性分子を含んだウィルスを投与しました そしてご覧の通り マウスは壁を避け 小さな台へとたどり着きます 目の知覚機能が回復しています またこのラットの凄いところは 失明を経験していないラットと 同等の成績で台にたどり着いているということです この前臨床研究は 今後我々が実現したいことの 希望を示していると考えます
最後に我々はニューロテクノロジーの分野における 新たなビジネスモデルも模索していることをお伝えしておきます 我々はこういったものを開発し 皆が様々な障害の治療を研究できるよう 世界中で数百のグループに自由に共有しています 我々の望みは 修復および設計が出来る程度まで 脳の回路を解明し 先にお話しした不治の難病を 21 世紀で過去のものとすることです
ありがとうございました
(拍手)
ファン・エンリケズ: 少々 濃い内容でした (笑い) 薬の代わりに光で 発作やてんかんを抑え また治療ターゲットを 同定するという狙いが まず第一ですね 第二に 脳を二つの色でコントロールできるように なったとおっしゃっていたと思います スイッチのオンオフのように
エド・ボイデン: その通りです
JE: 脳内の全ての信号を二進数に置き換えられると
EB: そうですね 青い光では 1 として情報を促進させられます 光を弱めると大体 0 になります 障害者の機能を増強するために 我々は最終的に脳と作動する コプロセッサを作りたいと考えています
JE: つまり理論的には マウスが感じ 匂いをかぎ 聞き 触れるのを 1 と 0 の文字列でモデル化できるということですか?
EB: そうです 我々はこの技術を どの神経信号が特定の行動 考え 感情へと導くかの評価に用い 脳の解明に役立てたいと思います
JE: ではいつか記憶のダウンロードやアップロードが できるようになるということですか?
EB: ええ 我々はそれにも頑張って取り組み始めています 我々は現在 情報を外部記録し また取り入れられるように 記録素子を脳に敷き詰めようという研究も進めています 脳の情報処理を増強するのに必要なものを 計算しているといったところです
JE: それは色々変化をもたらすかもしれませんね ありがとうございました
(拍手)
品詞分類
- 主語
- 動詞
- 助動詞
- 準動詞
- 関係詞等
TED 日本語
TED Talks
関連動画
うつやPTSDを予防できる新種の薬 | TED Talkレベッカ・ブラックマン
2019.04.17騒音が健康に有害な理由 ― そして私たちにできることマティアス・バスナー
2019.02.26さまよう心を鎮めるには | TED Talkアミシ・ジャー
2018.04.18鬱の友達と心を通わせるにはビル・バーナット
2018.03.23脳に良い変化をもたらす運動の効果ウェンディー・スズキ
おすすめ 12018.03.21物事の「良し悪し」は思い込みに過ぎないヘザー・ラニエ
おすすめ 12018.01.19脳が深い睡眠から更なる恩恵を得る方法ダン・ガーテンバーグ
2018.01.04うつを一人で抱え込まないでニッキー・ウェバー・アレン
2017.10.26長寿の秘訣は周囲の人との交流かもスーザン・ピンカー
2017.09.04脳はどのように美しさを判定するか?アンジャン・チャタジー
2017.08.22脳が「意識された現実」という幻覚を作り出す仕組みアニル・セス
2017.07.18注意を向けた時、脳では何が起きているのかメディ・オディカニ=セイドラー
2017.07.12ティーンエイジャーの登校時間を遅らせるべき理由とはウェンディ・トロクセル
2017.06.09死に直面したとき、人生に生きる価値を与えてくれるのはルーシー・カラニシ
おすすめ 12017.06.07最善の自己と最悪の自己の生物学ロバート・サポルスキー
2017.05.31メンタルヘルスを気遣うのは恥ずかしくなんかないサング・デリ
2017.05.26
洋楽 おすすめ
RECOMMENDS
洋楽歌詞
ダイナマイトビーティーエス
洋楽最新ヒット2020.08.20ディス・イズ・ミーグレイテスト・ショーマン・キャスト
洋楽人気動画2018.01.11グッド・ライフGイージー、ケラーニ
洋楽人気動画2017.01.27ホワット・ドゥ・ユー・ミーン?ジャスティン・ビーバー
洋楽人気動画2015.08.28ファイト・ソングレイチェル・プラッテン
洋楽人気動画2015.05.19ラヴ・ミー・ライク・ユー・ドゥエリー・ゴールディング
洋楽人気動画2015.01.22アップタウン・ファンクブルーノ・マーズ、マーク・ロンソン
洋楽人気動画2014.11.20ブレイク・フリーアリアナ・グランデ
洋楽人気動画2014.08.12ハッピーファレル・ウィリアムス
ポップス2014.01.08カウンティング・スターズワンリパブリック
ロック2013.05.31ア・サウザンド・イヤーズクリスティーナ・ペリー
洋楽人気動画2011.10.26ユー・レイズ・ミー・アップケルティック・ウーマン
洋楽人気動画2008.05.30ルーズ・ユアセルフエミネム
洋楽人気動画2008.02.21ドント・ノー・ホワイノラ・ジョーンズ
洋楽人気動画2008.02.15オンリー・タイムエンヤ
洋楽人気動画2007.10.03ミス・ア・シングエアロスミス
ロック2007.08.18タイム・トゥ・セイ・グッバイサラ・ブライトマン
洋楽人気動画2007.06.08シェイプ・オブ・マイ・ハートスティング
洋楽人気動画2007.03.18ウィ・アー・ザ・ワールド(U.S.A. フォー・アフリカ)マイケル・ジャクソン
洋楽人気動画2006.05.14ホテル・カリフォルニアイーグルス
ロック2005.07.06