
TED日本語
TED Talks(英語 日本語字幕付き動画)
TED日本語 - モリー・スティーブンス: 新しい骨再生法
TED Talks
新しい骨再生法
A new way to grow bone
モリー・スティーブンス
Molly Stevens
内容
まとまった量の骨を再生するのに、何が必要なのでしょうか。典型的な骨の再生法では、患者の腰から採骨し、損傷した所に埋め込むのですが、限界もあり、手術後2、3年でかなりの痛みが生じうるのです。情報を豊かに織り込み、モリー・スティーブンスは、骨生来の能力を使い、痛みを伴わず骨組織の再生をする新しい幹細胞応用法を紹介します。
字幕
SCRIPT
Script
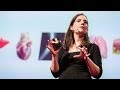
As humans, it's in our nature to want to improve our health and minimize our suffering. Whatever life throws at us, whether it's cancer, diabetes, heart disease, or even broken bones, we want to try and get better. Now I'm head of a biomaterials lab, and I'm really fascinated by the way that humans have used materials in really creative ways in the body over time.
Take, for example, this beautiful blue nacre shell. This was actually used by the Mayans as an artificial tooth replacement. We're not quite sure why they did it. It's hard. It's durable. But it also had other very nice properties. In fact, when they put it into the jawbone, it could integrate into the jaw, and we know now with very sophisticated imaging technologies that part of that integration comes from the fact that this material is designed in a very specific way, has a beautiful chemistry, has a beautiful architecture. And I think in many ways we can sort of think of the use of the blue nacre shell and the Mayans as the first real application of the bluetooth technology.
(Laughter)
But if we move on and think throughout history how people have used different materials in the body, very often it's been physicians that have been quite creative. They've taken things off the shelf.
One of my favorite examples is that of Sir Harold Ridley, who was a famous ophthalmologist, or at least became a famous ophthalmologist. And during World War II, what he would see would be pilots coming back from their missions, and he noticed that within their eyes they had shards of small bits of material lodged within the eye, but the very interesting thing about it was that material, actually, wasn't causing any inflammatory response. So he looked into this, and he figured out that actually that material was little shards of plastic that were coming from the canopy of the Spitfires. And this led him to propose that material as a new material for intraocular lenses. It's called PMMA, and it's now used in millions of people every year and helps in preventing cataracts.
And that example, I think, is a really nice one, because it helps remind us that in the early days, people often chose materials because they were bioinert. Their very purpose was to perform a mechanical function. You'd put them in the body and you wouldn't get an adverse response. And what I want to show you is that in regenerative medicine, we've really shifted away from that idea of taking a bioinert material. We're actually actively looking for materials that will be bioactive, that will interact with the body, and that furthermore we can put in the body, they'll have their function, and then they'll dissolve away over time.
If we look at this schematic, this is showing you what we think of as the typical tissue-engineering approach. We have cells there, typically from the patient. We can put those onto a material, and we can make that material very complex if we want to, and we can then grow that up in the lab or we can put it straight back into the patient. And this is an approach that's used all over the world, including in our lab.
But one of the things that's really important when we're thinking about stem cells is that obviously stem cells can be many different things, and they want to be many different things, and so we want to make sure that the environment we put them into has enough information so that they can become the right sort of specialist tissue. And if we think about the different types of tissues that people are looking at regenerating all over the world, in all the different labs in the world, there's pretty much every tissue you can think of. And actually, the structure of those tissues is quite different, and it's going to really depend on whether your patient has any underlying disease, other conditions, in terms of how you're going to regenerate your tissue, and you're going to need to think about the materials you're going to use really carefully, their biochemistry, their mechanics, and many other properties as well.
Our tissues all have very different abilities to regenerate, and here we see poor Prometheus, who made a rather tricky career choice and was punished by the Greek gods. He was tied to a rock, and an eagle would come every day to eat his liver. But of course his liver would regenerate every day, and so day after day he was punished for eternity by the gods. And liver will regenerate in this very nice way, but actually if we think of other tissues, like cartilage, for example, even the simplest nick and you're going to find it really difficult to regenerate your cartilage. So it's going to be very different from tissue to tissue.
Now, bone is somewhere in between, and this is one of the tissues that we work on a lot in our lab. And bone is actually quite good at repairing. It has to be. We've probably all had fractures at some point or other. And one of the ways that you can think about repairing your fracture is this procedure here, called an iliac crest harvest. And what the surgeon might do is take some bone from your iliac crest, which is just here, and then transplant that somewhere else in the body. And it actually works really well, because it's your own bone, and it's well vascularized, which means it's got a really good blood supply. But the problem is, there's only so much you can take, and also when you do that operation, your patients might actually have significant pain in that defect site even two years after the operation.
So what we were thinking is, there's a tremendous need for bone repair, of course, but this iliac crest-type approach really has a lot of limitations to it, and could we perhaps recreate the generation of bone within the body on demand and then be able to transplant it without these very, very painful aftereffects that you would have with the iliac crest harvest?
And so this is what we did, and the way we did it was by coming back to this typical tissue-engineering approach but actually thinking about it rather differently. And we simplified it a lot, so we got rid of a lot of these steps. We got rid of the need to harvest cells from the patient, we got rid of the need to put in really fancy chemistries, and we got rid of the need to culture these scaffolds in the lab. And what we really focused on was our material system and making it quite simple, but because we used it in a really clever way, we were able to generate enormous amounts of bone using this approach. So we were using the body as really the catalyst to help us to make lots of new bone. And it's an approach that we call the in vivo bioreactor, and we were able to make enormous amounts of bone using this approach. And I'll talk you through this.
So what we do is, in humans, we all have a layer of stem cells on the outside of our long bones. That layer is called the periosteum. And that layer is actually normally very, very tightly bound to the underlying bone, and it's got stem cells in it. Those stem cells are really important in the embryo when it develops, and they also sort of wake up if you have a fracture to help you with repairing the bone. So we take that periosteum layer and we developed a way to inject underneath it a liquid that then, within 30 seconds, would turn into quite a rigid gel and can actually lift the periosteum away from the bone. So it creates, in essence, an artificial cavity that is right next to both the bone but also this really rich layer of stem cells. And we go in through a pinhole incision so that no other cells from the body can get in, and what happens is that that artificial in vivo bioreactor cavity can then lead to the proliferation of these stem cells, and they can form lots of new tissue, and then over time, you can harvest that tissue and use it elsewhere in the body.
This is a histology slide of what we see when we do that, and essentially what we see is very large amounts of bone. So in this picture, you can see the middle of the leg, so the bone marrow, then you can see the original bone, and you can see where that original bone finishes, and just to the left of that is the new bone that's grown within that bioreactor cavity, and you can actually make it even larger. And that demarcation that you can see between the original bone and the new bone acts as a very slight point of weakness, so actually now the surgeon can come along, can harvest away that new bone, and the periosteum can grow back, so you're left with the leg in the same sort of state as if you hadn't operated on it in the first place. So it's very, very low in terms of after-pain compared to an iliac crest harvest. And you can grow different amounts of bone depending on how much gel you put in there, so it really is an on demand sort of procedure.
Now, at the time that we did this, this received a lot of attention in the press, because it was a really nice way of generating new bone, and we got many, many contacts from different people that were interested in using this. And I'm just going to tell you, sometimes those contacts are very strange, slightly unexpected, and the very most interesting, let me put it that way, contact that I had, was actually from a team of American footballers that all wanted to have double-thickness skulls made on their head.
And so you do get these kinds of contacts, and of course, being British and also growing up in France, I tend to be very blunt, and so I had to explain to them very nicely that in their particular case, there probably wasn't that much in there to protect in the first place.
(Laughter)
(Applause)
So this was our approach, and it was simple materials, but we thought about it carefully. And actually we know that those cells in the body, in the embryo, as they develop can form a different kind of tissue, cartilage, and so we developed a gel that was slightly different in nature and slightly different chemistry, put it in there, and we were able to get 100 percent cartilage instead.
And this approach works really well, I think, for pre-planned procedures, but it's something you do have to pre-plan. So for other kinds of operations, there's definitely a need for other scaffold-based approaches. And when you think about designing those other scaffolds, actually, you need a really multi-disciplinary team. And so our team has chemists, it has cell biologists, surgeons, physicists even, and those people all come together and we think really hard about designing the materials.
But we want to make them have enough information that we can get the cells to do what we want, but not be so complex as to make it difficult to get to clinic. And so one of the things we think about a lot is really trying to understand the structure of the tissues in the body. And so if we think of bone, obviously my own favorite tissue, we zoom in, we can see, even if you don't know anything about bone structure, it's beautifully organized, really beautifully organized. We've lots of blood vessels in there. And if we zoom in again, we see that the cells are actually surrounded by a 3D matrix of nano-scale fibers, and they give a lot of information to the cells. And if we zoom in again, actually in the case of bone, the matrix around the cells is beautifully organized at the nano scale, and it's a hybrid material that's part organic, part inorganic. And that's led to a whole field, really, that has looked at developing materials that have this hybrid kind of structure. And so I'm showing here just two examples where we've made some materials that have that sort of structure, and you can really tailor it. You can see here a very squishy one and now a material that's also this hybrid sort of material but actually has remarkable toughness, and it's no longer brittle. And an inorganic material would normally be really brittle, and you wouldn't be able to have that sort of strength and toughness in it.
One other thing I want to quickly mention is that many of the scaffolds we make are porous, and they have to be, because you want blood vessels to grow in there. But the pores are actually oftentimes much bigger than the cells, and so even though it's 3D, the cell might see it more as a slightly curved surface, and that's a little bit unnatural. And so one of the things you can think about doing is actually making scaffolds with slightly different dimensions that might be able to surround your cells in 3D and give them a little bit more information. And there's a lot of work going on in both of these areas.
Now finally, I just want to talk a little bit about applying this sort of thing to cardiovascular disease, because this is a really big clinical problem. And one of the things that we know is that, unfortunately, if you have a heart attack, then that tissue can start to die, and your outcome may not be very good over time. And it would be really great, actually, if we could stop that dead tissue either from dying or help it to regenerate. And there's lots and lots of stem cell trials going on worldwide, and they use many different types of cells, but one common theme that seems to be coming out is that actually, very often, those cells will die once you've implanted them. And you can either put them into the heart or into the blood system, but either way, we don't seem to be able to get quite the right number of cells getting to the location we want them to and being able to deliver the sort of beautiful cell regeneration that we would like to have to get good clinical outcomes.
And so some of the things that we're thinking of, and many other people in the field are thinking of, are actually developing materials for that. But there's a difference here. We still need chemistry, we still need mechanics, we still need really interesting topography, and we still need really interesting ways to surround the cells. But now, the cells also would probably quite like a material that's going to be able to be conductive, because the cells themselves will respond very well and will actually conduct signals between themselves. You can see them now beating synchronously on these materials, and that's a very, very exciting development that's going on.
So just to wrap up, I'd like to actually say that being able to work in this sort of field, all of us that work in this field that's not only super-exciting science, but also has the potential to impact on patients, however big or small they are, is really a great privilege. And so for that, I'd like to thank all of you as well.
Thank you.
(Applause)
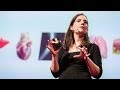
誰もが 健康を改善したり 肉体的苦しみを最小限に留め 我が身に起る事が 癌 糖尿病 心臓病または骨折と それが何であろうと 良くなりたいと思うものです 私は生体材料研究所の所長ですが 過去 様々な材料が 独創的な方法で人体に 使われてきたということに とても感心させられます
例えば この貝の青い真珠層をご覧下さい これはマヤ族が歯のインプラントに 実際 使っていました その理由はよく分かりませんが 硬く長持ちし 他にもとても良い特質があります 顎に入れると 顎骨と融合するのです 高度画像技術で 分かっている 融合の理由は この材料の 用途によく合ったデザイン そして素晴らしい化学的性質と 構造にありました あらゆる意味で マヤ族の青い真珠層を持つ貝の使い道は まさしく最初の ブルートゥース技術だ なんて思ったりします
(笑)
先に進んで 歴史を通して 人類が体に様々な種類の材料を 使ってきたことを考えてみると 創意工夫をしてきたのは 医師の場合が多く 彼らが様々な発明をしてきました
その中でも 私のお気に入りは サー・ハロルド・リドリーのものです 彼は有名な眼科医で ― 少なくとも そうなったのですが ― 第2次世界大戦中 彼は 戦線から戻って来たパイロットを見て 彼らの目の中に 小さな異物のかけらが 入っているのに気がつきました 興味深いことに その物質は炎症反応を 全く引き起こしていなかったのでした 調べて分かったことは その物質は 小さなプラスチックのかけらで スピットファイア戦闘機の天蓋から 来る物でした それで彼はこの物質を 新しい眼内レンズの素材 として提唱したのです PMMAと呼ばれるもので 毎年 何百万人の人の目に 白内障を防ぐ為に使われています
この例は 昔は機械的機能をさせる為に よく生体不活性材料が 選ばれ 使われていた ということを 示しています 生体不活性材料を体に入れても 拒絶反応はありません しかし ここで私が述べたい事は 再生医療は 生体不活性材料から 全く離れたということです 我々が積極的に探している材料は 生体と作用する 生体活性材料で 生体内に入れられると そこで機能し 時が経つにつれ 生体内に吸収されるものです
このチャートをご覧下さい これが示しているのは 細胞組織工学の 典型的アプローチです 普通患者から細胞を取り それを材料に入れ 非常に複雑なものに することもでき ― 実験室で増殖するか 患者の体に直接 戻すか どちらでもできます これが世界中で そして 我々の実験室でも 行われている方法です
幹細胞について 本当に大切なことの1つは 幹細胞は あらゆる組織に分化でき 又 そうなる傾向にあるので 幹細胞を入れる環境に 我々が必要な情報を 確実に組み込むと 目的の特定の組織に なるという事です 世界中の実験室で 再生が試みられている 組織のタイプは 殆ど考え得る全ての組織 と言っていい程です そんな組織の構造は かなり多様で 患者の他の 隠れた病気とか健康問題が 組織の再生法や 材料の使用法や 生化学的性質、 機能 その他多くの特質に影響し それにより 我々の対処法も大きく変わってきます
組織は其々 異なる再生能力があります ここで思い出すのが 可哀想なプロメテウス 危なっかしい決断をした彼は ギリシャの神々に罰せられ 岩に縛り付けられ 鷲が毎日 彼の肝臓をついばみに来ます 彼の肝臓は毎日再生し そうやって来る日も来る日も 永遠に神々に罰せられるのです 肝臓はこのように 再生されることになるでしょうが 他の組織 例えば軟骨は どんな些細な欠損でも 再生するのは とても難しいのです この様に組織により 違いが非常に大きく
骨の再生能力はその中間です 骨は我々の実験室で よく扱われる組織の1つで 自己修復能力は 実は かなり高いのです そうでなければ困ります たぶん我々はみな 骨折を経験しているでしょうし 骨折治療の1つの方法は 「腸骨採取」と呼ばれる手法で 外科医が 腸骨から骨を採取し ここにありますが ― 体の他の部分にそれを移植します これは本当にうまく行くのです 本人の骨なので うまく血管新生化し 血液の流れが とても良くなるのですが 問題は採骨できる量に 限界があるという事です その上 手術後 採骨した場所が 2年経っても 非常に痛む可能性があるのです
それで我々が考えた事は 勿論 骨修復の需要は 非常に大きいのですが ― 腸骨タイプのアプローチでは 限界があまりにあるので 必要に応じ 生体内で 骨を再生し 移植したらどうだろう それにより腸骨採取後のような 極度の痛みが伴わない移植が 出来るのではないだろうか? ということです
その我々のやり方は 典型的な細胞組織工学の アプローチに戻ったのですが かなり違った観点を取りました 随分 簡素化して かなりステップを省きました 患者からの 細胞採取の必要性 あらゆる高価な 化学薬品の必要性 そして研究室で担体を 培養する必要性を なくしました 我々が本当に 焦点を置いているのは 材料系と それを簡素化する事ですが よく考えられた方法で使用したので このアプローチによって 膨大な量の骨を 再生できたのです それで我々は生体を 骨を大量に作る為の 媒体として使いました そのアプローチを 「生体バイオリアクター」と呼び このやり方で とてつもない量の骨を 再生できるのです 分かり易く説明すると
こうです 生体には幹細胞の層が 長骨の外側にあり 「骨膜」とよばれ 普段とても しっかりと その下の骨に密着していて 幹細胞を含んでいます この幹細胞は 胎芽の成長にとても重要で 骨折すると 骨を修復しようと活性化します 我々はその骨膜に目をつけ その下に液体を 注入する方法を開発しました その液体は注入後30秒内で 固形のゲルになり 骨から骨膜を持ち上げる事ができ 人工の空洞が 骨と幹細胞豊かな骨膜の間にできます 微小な切り口から入るので 生体の他の細胞は入れません 人工的に作られた空洞 バイオリアクター・スペースが 幹細胞の増殖に繋がり 多くの新しい組織を作り 時が経つと組織を採取し 人体の他の場所に移植できます
これがそのプロセスで見られる 組織構造のスライドです 我々が見ているのは 非常に大量の骨です この写真では足の中の中央部 ― 骨髄が右端に見え 次に本来の骨があり その骨が終わった所の 丁度左に新生骨が バイオリアクター・スペース内で 再生しています もっと大きくもできます 本来の骨と新生骨との 境界部分は ほんの少しだけですが弱い所で ここが外科医の出番です 新生骨を採取し 骨膜が再生します 初めっから手術など しなかったような状態の 足が戻ってきます それで術後の痛みは 腸骨採集後と比べれば とても軽いのです 骨再生量は ゲルの注入量次第なので 必要に応じて調節できます
我々が これを公表した時 メディアの注目を浴びました 新しい骨再生の 実にいい方法だからです これを使いたいと言う 様々な人々から問い合わせが来ました 実のところ 全くおかしなものもありました 思ってもなかったような とっても面白いもの と言ったらいいでしょう その1つは 米国のフットボール選手からで 自分たちの頭蓋骨の厚みを 2倍にしたいというものでした
こんな問い合わせは 本当にあるのです フランスで育った イギリス人の私は ちょっと辛口な傾向があり 彼らにこう説明しました 「あなた達の様な特殊ケースでは たぶんその中にはあまり 守るものなんて ないんじゃないの」って
(笑)
(拍手)
これが我々のアプローチでした 単純な材料ですが 入念に計画しました 生体や胎芽の幹細胞が 成長すると ある異なる組織の 軟骨になるのが分かっているので 少し化学的性質の違うゲルを開発し それを注入し軟骨を100% 再生できました
これはあらかじめ計画されると とてもうまく行くやり方で 前もって計画しなければ ならないものです 他の手術では 他の担体を基礎とした アプローチが確かに必要です 他の担体を開発する時は 様々な分野からの 専門家チームが必要です 我々のチームには化学者 細胞生物学者 外科医 そして物理学者さえもが加わり 皆で一体となり 材料の開発に懸命に 取り組んでいます
特定の細胞機能をさせるよう また実用化に向け複雑にならないよう 材料には 十分な情報を 備えて欲しいのです 我々の仕事の1つは 生体組織構造の理解を 深める事です 骨について考えると 私が関心を持つ組織ですが ― ズームインしてみると 皆さんが骨組織の事は よく知らなくても 本当に素晴らしく 組織化されていると分かります そこには血管が張り巡っています 更にズームインすると ナノスケール繊維の 3D基質が細胞を囲み 細胞に多くの情報を与えています 更にズームインすると 骨の場合 細胞周辺の基質は美しく ナノスケールで纏まり 混成された 有機・無機質のハイブリッドです ここで全く新しい分野 ハイブリッド的な構造を持った 材料の開発分野に移り その例を2つだけお見せします ハイブリッド的構造を持った 調整可能な材料を作りました ここにブヨブヨした物が見えますが これもハイブリッド的材料で 驚く程強く 壊れ易くありません 無機質の材料は通常とても脆く このような丈夫さや強さはありません
もう1つだけちょっと 触れておきたい事は 我々が作る担体の多くは そこに血管が通り 育つように多孔質です 穴のサイズは 細胞より大きく 3Dではありますが 細胞には少しカーブした表面のように 見えるかもしれませんね 少々不自然です ですから 考えないといけないのは 担体を少し違う次元で 3Dで細胞を包み もう少し情報を 細胞に与えるように作る事です これらの領域では 色々な研究がなされています
最後に少しだけ これを心血管疾患に応用する事 についてお話します 実に大きな臨床上の問題だからです 分かっている事の1つは 残念ながら 心臓発作を起こしたら 組織は活動停止し始めるため 見通しは 時と共に 悪くなって行くかもしれません 我々が 組織の壊死を 阻止するか 再生を促すか どちらか出来るなら どんなにか素晴らしいことでしょう 今 世界中で行われている 幹細胞を使った臨床試験では あるゆるタイプの 細胞を使っていますが 表面化してきた共通の課題は 細胞は心臓に移植されると 死んでしまう事が多い ということです 細胞を心臓か 血液システムに 入れる事はできますが どちらにしろ 十分な数の細胞を 正しい場所に入れ 思わしい臨床結果が得られる 細胞再生ができないようなのです 思わしい臨床結果が得られる 細胞再生ができないようなのです
我々とこの分野の人々は その問題を解決する為の材料を 開発する事を考えています しかし1つ違いがあります その為には我々は 化学的技術的な向上 参考となるトポグラフィー画像 適切な細胞を囲む方法がまだ必要です 細胞はまた 伝導体のような働きを するようになるでしょう なぜなら細胞そのものは よく反応し お互い同士でシグナルを 伝え合うからです 今でも このように 材料の中で細胞は 同調し合い脈打っています とても興奮させられる事が 起きています
最後に この分野で働く我々に取って とても夢のある科学分野であり 大なり小なり患者にインパクトを 与える可能性のあるこの分野で 働けるという事を 本当に光栄に思います その為にも 皆様にも感謝を述べたいと思います
ありがとうございます
(拍手)
品詞分類
- 主語
- 動詞
- 助動詞
- 準動詞
- 関係詞等
TED 日本語
TED Talks
関連動画
うつやPTSDを予防できる新種の薬 | TED Talkレベッカ・ブラックマン
2019.04.17騒音が健康に有害な理由 ― そして私たちにできることマティアス・バスナー
2019.02.26さまよう心を鎮めるには | TED Talkアミシ・ジャー
2018.04.18鬱の友達と心を通わせるにはビル・バーナット
2018.03.23脳に良い変化をもたらす運動の効果ウェンディー・スズキ
おすすめ 12018.03.21物事の「良し悪し」は思い込みに過ぎないヘザー・ラニエ
おすすめ 12018.01.19脳が深い睡眠から更なる恩恵を得る方法ダン・ガーテンバーグ
2018.01.04うつを一人で抱え込まないでニッキー・ウェバー・アレン
2017.10.26長寿の秘訣は周囲の人との交流かもスーザン・ピンカー
2017.09.04脳はどのように美しさを判定するか?アンジャン・チャタジー
2017.08.22脳が「意識された現実」という幻覚を作り出す仕組みアニル・セス
2017.07.18注意を向けた時、脳では何が起きているのかメディ・オディカニ=セイドラー
2017.07.12ティーンエイジャーの登校時間を遅らせるべき理由とはウェンディ・トロクセル
2017.06.09死に直面したとき、人生に生きる価値を与えてくれるのはルーシー・カラニシ
おすすめ 12017.06.07最善の自己と最悪の自己の生物学ロバート・サポルスキー
2017.05.31メンタルヘルスを気遣うのは恥ずかしくなんかないサング・デリ
2017.05.26
洋楽 おすすめ
RECOMMENDS
洋楽歌詞
ステイザ・キッド・ラロイ、ジャスティン・ビーバー
洋楽最新ヒット2021.08.20スピーチレス~心の声ナオミ・スコット
洋楽最新ヒット2019.05.23シェイプ・オブ・ユーエド・シーラン
洋楽人気動画2017.01.30フェイデッドアラン・ウォーカー
洋楽人気動画2015.12.03ウェイティング・フォー・ラヴアヴィーチー
洋楽人気動画2015.06.26シー・ユー・アゲインウィズ・カリファ
洋楽人気動画2015.04.06シュガーマルーン5
洋楽人気動画2015.01.14シェイク・イット・オフテイラー・スウィフト
ポップス2014.08.18オール・アバウト・ザット・ベースメーガン・トレイナー
ポップス2014.06.11ストーリー・オブ・マイ・ライフワン・ダイレクション
洋楽人気動画2013.11.03コール・ミー・メイビーカーリー・レイ・ジェプセン
洋楽人気動画2012.03.01美しき生命コールドプレイ
洋楽人気動画2008.08.04バッド・デイ~ついてない日の応援歌ダニエル・パウター
洋楽人気動画2008.05.14サウザンド・マイルズヴァネッサ・カールトン
洋楽人気動画2008.02.19イッツ・マイ・ライフボン・ジョヴィ
ロック2007.10.11アイ・ウォント・イット・ザット・ウェイバックストリート・ボーイズ
洋楽人気動画2007.09.14マイ・ハート・ウィル・ゴー・オンセリーヌ・ディオン
洋楽人気動画2007.07.12ヒーローマライア・キャリー
洋楽人気動画2007.03.21オールウェイズ・ラヴ・ユーホイットニー・ヒューストン
洋楽人気動画2007.02.19オネスティビリー・ジョエル
洋楽人気動画2005.09.16