
TED日本語
TED Talks(英語 日本語字幕付き動画)
TED日本語 - アンソニー・アタラ: 臓器の培養
TED Talks
臓器の培養
Anthony Atala on growing organs
アンソニー・アタラ
内容
アンソニー・アタラの最先端技術を駆使した研究所では筋肉、血管、膀胱を始め様々な人間の臓器が培養されています。TEDMEDでアタラ医師は生体工学の研究者たちと取り組んでいる様子を紹介します。摂氏37度に予熱されるオーブンのようなバイオリアクタ―や人間の組織をプリントする機械など、どれもSF小説の中から飛び出して来たような発明ばかりです。
字幕
SCRIPT
Script
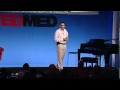
This is actually a painting that hangs at the Countway Library at Harvard Medical School. And it shows the first time an organ was ever transplanted. In the front, you see, actually, Joe Murray getting the patient ready for the transplant, while in the back room you see Hartwell Harrison, the Chief of Urology at Harvard, actually harvesting the kidney. The kidney was indeed the first organ ever to be transplanted to the human.
That was back in 1954,55 years ago. Yet we're still dealing with a lot of the same challenges as many decades ago. Certainly many advances, many lives saved. But we have a major shortage of organs. In the last decade the number of patients waiting for a transplant has doubled. While, at the same time, the actual number of transplants has remained almost entirely flat. That really has to do with our aging population. We're just getting older. Medicine is doing a better job of keeping us alive. But as we age, our organs tend to fail more.
So, that's a challenge, not just for organs but also for tissues. Trying to replace pancreas, trying to replace nerves that can help us with Parkinson's. These are major issues. This is actually a very stunning statistic. Every 30 seconds a patient dies from diseases that could be treated with tissue regeneration or replacement. So, what can we do about it? We've talked about stem cells tonight. That's a way to do it. But still ways to go to get stem cells into patients, in terms of actual therapies for organs.
Wouldn't it be great if our bodies could regenerate? Wouldn't it be great if we could actually harness the power of our bodies, to actually heal ourselves? It's not really that foreign of a concept, actually; it happens on the Earth every day. This is actually a picture of a salamander. Salamanders have this amazing capacity to regenerate. You see here a little video. This is actually a limb injury in this salamander. And this is actually real photography, timed photography, showing how that limb regenerates in a period of days. You see the scar form. And that scar actually grows out a new limb.
So, salamanders can do it. Why can't we? Why can't humans regenerate? Actually, we can regenerate. Your body has many organs and every single organ in your body has a cell population that's ready to take over at the time of injury. It happens every day. As you age, as you get older. Your bones regenerate every 10 years. Your skin regenerates every two weeks. So, your body is constantly regenerating. The challenge occurs when there is an injury. At the time of injury or disease, the body's first reaction is to seal itself off from the rest of the body. It basically wants to fight off infection, and seal itself, whether it's organs inside your body, or your skin, the first reaction is for scar tissue to move in, to seal itself off from the outside.
So, how can we harness that power? One of the ways that we do that is actually by using smart biomaterials. How does this work? Well, on the left side here you see a urethra which was injured. This is the channel that connects the bladder to the outside of the body. And you see that it is injured. We basically found out that you can use these smart biomaterials that you can actually use as a bridge. If you build that bridge, and you close off from the outside environment, then you can create that bridge, and cells that regenerate in your body, can then cross that bridge, and take that path.
That's exactly what you see here. It's actually a smart biomaterial that we used, to actually treat this patient. This was an injured urethra on the left side. We used that biomaterial in the middle. And then,six months later on the right-hand side you see this reengineered urethra. Turns out your body can regenerate, but only for small distances. The maximum efficient distance for regeneration is only about one centimeter. So, we can use these smart biomaterials but only for about one centimeter to bridge those gaps.
So, we do regenerate, but for limited distances. What do we do now, if you have injury for larger organs? What do we do when we have injuries for structures which are much larger than one centimeter? Then we can start to use cells. The strategy here, is if a patient comes in to us with a diseased or injured organ, you can take a very small piece of tissue from that organ, less than half the size of a postage stamp, you can then tease that tissue apart, and look at its basic components, the patient's own cells, you take those cells out, grow and expand those cells outside the body in large quantities, and then we then use scaffold materials.
To the naked eye they look like a piece of your blouse, or your shirt, but actually these materials are fairly complex and they are designed to degrade once inside the body. It disintegrates a few months later. It's acting only as a cell delivery vehicle. It's bringing the cells into the body. It's allowing the cells to regenerate new tissue, and once the tissue is regenerated the scaffold goes away.
And that's what we did for this piece of muscle. This is actually showing a piece of muscle and how we go through the structures to actually engineer the muscle. We take the cells, we expand them, we place the cells on the scaffold, and we then place the scaffold back into the patient. But actually, before placing the scaffold into the patient, we actually exercise it. We want to make sure that we condition this muscle, so that it knows what to do once we put it into the patient. That's what you're seeing here. You're seeing this muscle bio-reactor actually exercising the muscle back and forth.
Okay. These are flat structures that we see here, the muscle. What about other structures? This is actually an engineered blood vessel. Very similar to what we just did, but a little bit more complex. Here we take a scaffold, and we basically -- scaffold can be like a piece of paper here. And we can then tubularize this scaffold. And what we do is we, to make a blood vessel, same strategy. A blood vessel is made up of two different cell types. We take muscle cells, we paste, or coat the outside with these muscle cells, very much like baking a layer cake, if you will.
You place the muscle cells on the outside. You place the vascular blood vessel lining cells on the inside. You now have your fully seeded scaffold. You're going to place this in an oven-like device. It has the same conditions as a human body,37 degrees centigrade,95 percent oxygen. You then exercise it, as what you saw on that tape.
And on the right you actually see a carotid artery that was engineered. This is actually the artery that goes from your neck to your brain. And this is an X-ray showing you the patent, functional blood vessel. More complex structures such as blood vessels, urethras, which I showed you, they're definitely more complex because you're introducing two different cell types. But they are really acting mostly as conduits. You're allowing fluid or air to go through at steady states. They are not nearly as complex as hollow organs. Hollow organs have a much higher degree of complexity, because you're asking these organs to act on demand.
So, the bladder is one such organ. Same strategy, we take a very small piece of the bladder, less than half the size of a postage stamp. We then tease the tissue apart into its two individual cell components, muscle, and these bladder specialized cells. We grow the cells outside the body in large quantities. It takes about four weeks to grow these cells from the organ. We then take a scaffold that we shape like a bladder. We coat the inside with these bladder lining cells. We coat the outside with these muscle cells. We place it back into this oven-like device. From the time you take that piece of tissue,six to eight weeks later you can put the organ right back into the patient.
This actually shows the scaffold. The material is actually being coated with the cells. When we did the first clinical trial for these patients we actually created the scaffold specifically for each patient. We brought patients in,six to eight weeks prior to their scheduled surgery, did X-rays, and we then composed a scaffold specifically for that patient's size pelvic cavity. For the second phase of the trials we just had different sizes, small, medium, large and extra-large. (Laughter) It's true. And I'm sure everyone here wanted an extra-large. Right? (Laughter)
So, bladders are definitely a little bit more complex than the other structures. But there are other hollow organs that have added complexity to it. This is actually a heart valve, which we engineered. And the way you engineer this heart valve is the same strategy. We take the scaffold, we seed it with cells, and you can now see here, the valve leaflets opening and closing. We exercise these prior to implantation. Same strategy.
And then the most complex are the solid organs. For solid organs, they're more complex because you're using a lot more cells per centimeter. This is actually a simple solid organ like the ear. It's now being seeded with cartilage. That's the oven-like device; once it's coated it gets placed there. And then a few weeks later we can take out the cartilage scaffold.
This is actually digits that we're engineering. These are being layered,one layer at a time, first the bone, we fill in the gaps with cartilage. We then start adding the muscle on top. And you start layering these solid structures. Again, fairly more complex organs, but by far, the most complex solid organs are actually the vascularized, highly vascularized, a lot of blood vessel supply, organs such as the heart, the liver, the kidneys. This is actually an example -- several strategies to engineer solid organs.
This is actually one of the strategies. We use a printer. And instead of using ink, we use -- you just saw an inkjet cartridge -- we just use cells. This is actually your typical desktop printer. It's actually printing this two chamber heart,one layer at a time. You see the heart coming out there. It takes about 40 minutes to print, and about four to six hours later you see the muscle cells contract. (Applause) This technology was developed by Tao Ju, who worked at our institute. And this is actually still, of course, experimental, not for use in patients.
Another strategy that we have followed is actually to use decellularized organs. We actually take donor organs, organs that are discarded, and we then can use very mild detergents to take all the cell elements out of these organs. So, for example on the left panel, top panel, you see a liver. We actually take the donor liver, we use very mild detergents, and we, by using these mild detergents, we take all the cells out of the liver.
Two weeks later, we basically can lift this organ up, it feels like a liver, we can hold it like a liver, it looks like a liver, but it has no cells. All we are left with is the skeleton, if you will, of the liver, all made up of collagen, a material that's in our bodies, that will not reject. We can use it from one patient to the next. We then take this vascular structure and we can prove that we retain the blood vessel supply.
You can see, actually that's a fluoroscopy. We're actually injecting contrast into the organ. Now you can see it start. We're injecting the contrast into the organ into this decellularized liver. And you can see the vascular tree that remains intact. We then take the cells, the vascular cells, blood vessel cells, we perfuse the vascular tree with the patient's own cells. We perfuse the outside of the liver with the patient's own liver cells. And we can then create functional livers. And that's actually what you're seeing. This is still experimental. But we are able to actually reproduce the functionality of the liver structure, experimentally.
For the kidney, as I talked to you about the first painting that you saw, the first slide I showed you,90 percent of the patients on the transplant wait list are waiting for a kidney,90 percent. So, another strategy we're following is actually to create wafers that we stack together, like an accordion, if you will. So, we stack these wafers together, using the kidney cells. And then you can see these miniature kidneys that we've engineered. They are actually making urine. Again, small structures, our challenge is how to make them larger, and that is something we're working on right now at the institute. One of the things that I wanted to summarize for you then is what is a strategy that we're going for in regenerative medicine.
If at all possible, we really would like to use smart biomaterials that we can just take off the shelf and regenerate your organs. We are limited with distances right now, but our goal is actually to increase those distances over time. If we can not use smart biomaterials, then we'd rather use your very own cells.
Why? Because they will not reject. We can take cells from you, create the structure, put it right back into you, they will not reject. And if possible, we'd rather use the cells from your very specific organ. If you present with a diseased wind pipe we'd like to take cells from your windpipe. If you present with a diseased pancreas we'd like to take cells from that organ.
Why? Because we'd rather take those cells which already know that those are the cell types you want. A windpipe cell already knows it's a windpipe cell. We don't need to teach it to become another cell type. So, we prefer organ-specific cells. And today we can obtain cells from most every organ in your body, except for several which we still need stem cells for, like heart, liver, nerve and pancreas. And for those we still need stem cells. If we can not use stem cells from your body then we'd like to use donor stem cells. And we prefer cells that will not reject and will not form tumors.
And we're working a lot with the stem cells that we published on two years ago, stem cells from the amniotic fluid, and the placenta, which have those properties. So, at this point, I do want to tell you that some of the major challenges we have. You know, I just showed you this presentation, everything looks so good, everything works. Actually no, these technologies really are not that easy. Some of the work you saw today was performed by over 700 researchers at our institute across a 20-year time span.
So, these are very tough technologies. Once you get the formula right you can replicate it. But it takes a lot to get there. So, I always like to show this cartoon. This is how to stop a runaway stage. And there you see the stagecoach driver, and he goes, on the top panel, He goes A, B, C, D, E, F. He finally stops the runaway stage. And those are usually the basic scientists, The bottom is usually the surgeons. (Laughter) I'm a surgeon so that's not that funny. (Laughter)
But actually method A is the correct approach. And what I mean by that is that anytime we've launched one of these technologies to the clinic, we've made absolutely sure that we do everything we can in the laboratory before we ever launch these technologies to patients. And when we launch these technologies to patients we want to make sure that we ask ourselves a very tough question. Are you ready to place this in your own loved one, your own child, your own family member, and then we proceed. Because our main goal, of course, is first, to do no harm.
I'm going to show you now, a very short clip, It's a five second clip of a patient who received one of the engineered organs. We started implanting some of these structures over 14 years ago. So, we have patients now walking around with organs, engineered organs, for over 10 years, as well. I'm going to show a clip of one young lady. She had a spina bifida defect, a spinal cord abnormality. She did not have a normal bladder. This is a segment from CNN. We are just taking five seconds. This is a segment that Sanjay Gupta actually took care of.
Video: Kaitlyn M: I'm happy. I was always afraid that I was going to have like, an accident or something. And now I can just go and go out with my friends, go do whatever I want.
Anthony Atala: See, at the end of the day, the promise of regenerative medicine is a single promise. And that is really very simple, to make our patients better. Thank you for your attention. (Applause)
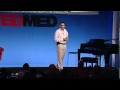
これはハーバード大学医学部の 図書館に飾ってある絵画です 初めての臓器移植の様子が描かれています ジョー・ミュレーの様子が伺えます そして 後ろの部屋には ハーバードの泌尿器科科長である ハートウェル・ハリソンが腎臓を 摘出している様子が描かれています 実に腎臓こそが人間に移植された 最初の臓器なのです
それは1954年のことでした 55年も前の話です 我々は何十年も経った今も 同じ難題に挑戦しています もちろん たくさんの医学的進歩があり たくさんの命が救われました しかし 私たちは重大な臓器の不足に直面しています 臓器移植の順番を待つ患者は ここ十年で2倍に増えています その一方で臓器移植の 手術数はほとんど増えていません これは高齢化が関っているためで 寿命が延びているからです 医学の進歩によって 私たちは長生きできているのです しかし 私たちが年を重ねるとともに 私たちの臓器は衰えてゆくのです
これは私たちに課せられた難題です 臓器だけではなく組織も衰えて行きます 膵臓の移植や パーキンソン病を和らげるための神経の移植に挑戦しています これらには重大な問題が伴います 驚くべき統計データがあるのですが 30秒に1人の割合で 体の一部を再生させたり 移植をすれば助かる患者が亡くなっているのです では私たちに何ができるのでしょうか? 今晩は幹細胞についてお話しています それこそ私たちが取るべき手段なのです しかし 幹細胞治療を臨床応用するには 長い道のりがあります
私たちの体を再生させられたら素晴らしいと思いませんか? もし 私たち自身の体の力を活用して 回復させることが実際にできたら素晴らしいと思いませんか? これは日常からかけ離れた概念なのではなく 実は地球上で日々起こっていることなのです これはサンショウウオの写真です サンショウウオはこのように驚くべき再生能力を持っています ビデオでご覧になっているのは 損傷したサンショウウオの足です 実際に サンショウウオの足が 数日のうちに再生する様子を 時間を追って撮影した写真です 瘢痕ができているのが見えますね 瘢痕の部分から 新たな足が伸びてきます
サンショウウオにはこのような事が出来るのです なぜ人間は再生機能がないのかと思うでしょうが 我々も再生する力をもっています 皆さんの体にはたくさん臓器があり それぞれの臓器には 傷ついたときに働きだす 細胞群が備わっており 休みなく働いています 皆さんが年をとるにつれて 皆さんの骨は10年ごとに再生されています 皆さんの皮膚も2週間ごとに再生されています 皆さんの体は常に再生され続けているのです 体が傷ついたとき その再生の挑戦が始まるのです 怪我をしたり 病気になると まず体は傷や病気が ほかの部分に広がらないように封じ込めようとします 体の他の部分へ感染が広がらないよう 封じ込めようとします それは臓器であれ皮膚であれ 最初の反応は外部と遮断するために 瘢痕組織がつくられます ではこの傷を封じ込めようとする力を
利用する方法はあるのでしょうか? 現に 高性能な生体材料を用いて 実用化されているのがその一つです どのように役立つのか見てみましょう 左の写真は損傷した尿道です これが膀胱から体の外につながる管です このように傷ついています まず その高性能な生体材料を 橋(ブリッジ)のように利用できることを発見しました ブリッジをつくり 外部の環境から封じ込めることができれば 体内で再生させた細胞に ブリッジを渡らせて 尿道をたどらせることが できるのです
これがその様子です これが 私たちが実際にこの患者の治療に用いた 高性能の生体材料です 左に見えるのは損傷した尿道です 中央に示している生体材料を用いました そして 6ヶ月後・・・右に見えるのが 再生された尿道です 短い間隔に限ってだけですが 私たちの体は再生できるのです 1cmまでしか 再生できないと言われています 高性能生体材料でも 1cmまでしか カバーできないのです
私たちは短い間隔であれば再生することができるのです より大きな臓器が損傷した場合 どうすれば良いのでしょうか 組織の損傷が 1cm以上の場合 どうすれば良いのでしょうか? このような時には細胞の出番です ここで取る戦略は次のようなものです 患者が臓器に問題を抱えて来院します その患者の臓器から一部を採取します 切手の半分以下の大きさです そして組織を細かく切って 基本的な成分を観察し 患者の細胞を 取り出して 患者の体外で培養させます そしてここで 臓器の骨組みとなる物質を用いるのです
裸眼では洋服の切れ端のように見えますが 実はこの物質は きわめて複雑で 体内に入ると分解されてしまいます 数カ月後には分解されてしまいます つまり 細胞を適切な場所に行き渡らせるためのみ働くのです 体内に細胞を運んで 新しい組織を再生できるようにします そして組織が再生されると骨組みは消えてしまいます
筋肉に施した方法をお見せします これは筋組織の一部と その構造のつくり方を示しています 細胞を取り出し増やした後に 細胞を骨組みに乗せ その骨組みを患者の体に戻します しかし その骨組みを患者の体に戻す前に できたての筋肉に運動をさせるのです 筋組織が患者の体内で 本来の動きができるように 運動させておくのです その運動がこれです 筋肉バイオリアクターが 筋肉を前後に運動させている様子をご覧いただいています
この平らな組織が 筋肉です それでは他の組織ではどうでしょうか? これは再生医療によってつくられた人工血管です 尿道をつくる工程と似ていますが もう少し複雑です まず骨組みを準備します 骨組みはまるで一枚の紙のようです それを筒状にして 血管も同じ方法でつくります 血管は2種類の細胞で構成されています 筋肉の細胞を取り出し 年輪のように重なるケーキを焼くように 筒の外側に筋細胞を
貼りつけていきます 内側には血管内皮細胞を配置します 細胞が植え付けられた骨組みを オーブンのような装置に入れます 人間の体内環境と同じで 摂氏37度 酸素95%にしてあります 先ほどのビデオのように 血管を動かします
右にみえるのは人工頸動脈です 頸動脈は首から脳につながる動脈です このレントゲン写真では きちんと機能している血管が見えます 血管や尿道などは もっと複雑な組織です なぜなら2種の異なる細胞を 導入することになるからです しかし 実際にこれらは導管としての役割を果たしています 液体か空気が 一定の状態で流れるようになっているのです 管状の組織は 空洞の臓器ほど複雑ではありません 空洞の臓器は状況に応じて機能するからです
膀胱もそのような臓器のひとつです 同様に膀胱から切手の半分以下の 大きさを切り取り その組織を分けて 筋肉と膀胱に特化した 二つの細胞成分に分類します 体外で細胞を大量に増やします 臓器から取り出した細胞は約4週間で培養できます そして骨組みを膀胱のような形に整えます 膀胱の内面を内側用の細胞で覆います そして外側を筋肉の細胞で覆います そしてオーブンのような装置へ戻します 組織の一部を採取してから6~8週間後に 臓器を患者の体に戻す事ができます
これは実際に骨組みを表したものです この物質は細胞で覆ってあります 臨床実験を最初に行った時は 各患者に合わせた骨組みを作りました 手術の6~8週間前に 患者に来てもらい レントゲン撮影の後 患者の骨盤腔の大きさにあった 骨組みを特別につくりました そして臨床試験の第2期には 小 中 大 特大サイズで骨組みをつくりました (聴衆より笑い) 本当です 皆さんは特大がいいんでしょう? (聴衆より笑い)
膀胱は明らかに他の組織よりも 少々複雑なのです さらに複雑な空洞の臓器もあります これは私たちが再生医療でつくった人工心臓弁です 心臓弁の作り方は同様の方法です 骨組みに細胞をのせます ご覧のとおり 弁が開いたり閉じたりしています 同じ方法を用いて移植の前に これらの臓器に運動をさせます
そして最も複雑なのは固形臓器です 固形臓器がより複雑なのは 1cmあたりに必要な細胞が数段に多いからです 例えば 耳です 軟骨細胞をのせているところです 細胞で覆った後は オーブンのような装置に 入れます そして数週間後 軟骨の骨組みを取り外す事ができます
指をつくっているところです これは一層 一層 積み重ねられています 最初に骨 そして隙間を軟骨でふさぎ 筋肉を上にのせて 固形組織の層を積み重ねます 繰り返しますが かなり複雑な臓器です しかし 他とは比べ物にならない複雑な固形臓器は 血管が多い臓器です 血管が多いのは 心臓 肝臓 そして腎臓などの 臓器です 固形臓器をつくる方法はいくつかありますが これはその一例です
私たちはプリンターを使います インクを使うのではなく 細胞を使います これは普通のプリンターです これは実は心臓の二つの小室を 一層ずつプリントしているのです ご覧になっているのは心臓で 約40分で印刷が終了し 4時間から6時間後には 筋肉が収縮し始めます (拍手) 私達の研究所にいたタオ・ジュ氏が開発した技術です この技術は 試験的なもので まだ患者に使用できる段階ではありません
また 脱細胞化した臓器も 使用しました ドナー提供された臓器を用いて 刺激の少ない洗浄剤で すべての細胞成分を これらの臓器から取り出します 左手のパネルと 上のパネルは肝臓です ドナー提供された肝臓を 洗浄します 刺激の少ない洗浄剤で すべての細胞を 肝臓から取り出すのです
2週間後にはこの臓器を持ち上げる事ができます 見た目も感触も 本物の肝臓のようですが 細胞はありません 肝臓を取り除くと コラーゲン製の骨組みが残ります コラーゲンは拒絶反応を 起こすことがありません これは何人もの患者に使用できます そしてこの血管組織を取り出し 血管の供給の維持を証明できます
これはフローラスコピーです ご覧になっているのは 造影剤を脱細胞化した肝臓に 注入しているところです 無傷のままの血管樹が見えます そこで 木のように枝分かれした血管に 患者自身の細胞を行き渡らせます 肝臓の外側に 患者自身の肝臓の細胞を 一面に散布します 肝臓を機能させるようにします ご覧になっているのが それです まだ試験段階ですが肝臓機能の再生を 成功させています
腎臓に話を移します 講演の最初にお見せした絵でも 腎臓移植をしていましたが 臓器移植が必要な患者の90%は 腎臓移植が必要な方たちです 私たちが試みているもう一つの方法は ウェハーをつくり それをアコーディオンのように積み重ねる方法です 腎臓の細胞を使ってウェハーを重ねます これは私たちがつくった小さな腎臓です これらは実は尿をつくり出しているのです 小さな組織をどうやって大きくするかという難題に 今 私達の研究所で 取り組んでいます 皆さんにお話ししたかった事のひとつは 再生医療が取ろうとしている戦略です
もし可能ならば 既製の高性能な生体材料を使って 患者の臓器を 再生させたいと思っています 再生できる幅は限られていますが 目標は その大きさを広げていくことです 高性能な生体材料の使用がだめなら 自分の細胞を使うことです
拒絶反応を起こさないからです 皆さんから細胞を取り出し組織をつくり出す そして それをそのまま皆さんに返すそうすれば拒絶反応は起きません もし可能なら 特定の臓器の細胞が好ましいのです 気管に問題がある場合は 気管の細胞を取り出します 膵臓に問題がある場合は 膵臓の細胞を取り出すわけです
理由は 採取する細胞は 自らが何の細胞なのか わかっているからです 気管の細胞は自身が気管の細胞だとわかっているので どんな細胞になるのか教える必要がありません よって特定の臓器の細胞がいいのです 今では 大半の臓器から細胞を得ることができますが 心臓 肝臓 神経 膵臓などがその例外で それらには依然として 幹細胞が必要です もし患者自身の幹細胞を使えない場合は ドナーの幹細胞を使います 拒絶反応を起こさず 腫瘍を形成しない細胞を選びます
そのような特性をもっているのは 2年前に発表した幹細胞です その幹細胞とは 羊水や胎盤から採取でき 私たちはその研究に取り組んでいます いくつかの難題についてお話したいと思います 紹介した技術は順調に見えますが 現実の答えはノーなのです それほど簡単ではありません 今日 紹介した内容の一部は 我々の研究所で700人以上の研究者が 20年の年月を経て 成し遂げたものです
とても難しい技術であって 正しい方式を見出すまで 道のりは長いのです ぜひこの漫画を見てください 暴走した馬車の阻止の仕方です 馬車の御者が上の絵では 馬の背をたどって 先頭の馬まで進んでいき 最後に暴走した馬車を阻止します 科学者なら そうするでしょう 下にいるのは通常 外科医です (聴衆より笑い) 外科医の私には面白くありません (聴衆より笑い)
しかし 実は上の方法が正しい取り組み方なのです それが意味するのは このような技術を 病院で患者に着手する時は どんな時でも絶対に研究室でやれる事の すべてをやってからにするよう 徹底しています また これらの技術を患者に施す前に 自らにとても難しい問いかけをするように徹底しています 自分の大切な人に この臓器移植をしても差し支えないかを問い その答えがイエスなら事を進めます なぜなら第一の目標は 危害を与えないことだからです
では とても短いのですが 培養した臓器の移植を受けた患者の ビデオをお見せします このような組織の移植は 14年以上前に始めました ですからこのような人工臓器を抱えて 10年以上元気に歩きまわっている患者がいるというわけです これからお見せする若い女性患者は 脊髄異常が原因の二分脊髄症で苦しんでいました 彼女の膀胱は正常ではなかった これはCNNニュースからのひとコマです 5秒間だけお時間頂きます これはサンジェイ・グプタが実際に携わったひとコマです
幸せです 私はいつも事故か何かで どうかなることを恐れていました でも 今は友達と 外へ繰り出し やりたい事ができるのです
結局のところ 再生医療の将来性が 唯一の希望なのです 本当にとても簡単ですが それだけで患者に勇気を与える事ができるのです ご清聴ありがとうございました (拍手)
品詞分類
- 主語
- 動詞
- 助動詞
- 準動詞
- 関係詞等
TED 日本語
TED Talks
関連動画
うつやPTSDを予防できる新種の薬 | TED Talkレベッカ・ブラックマン
2019.04.17騒音が健康に有害な理由 ― そして私たちにできることマティアス・バスナー
2019.02.26さまよう心を鎮めるには | TED Talkアミシ・ジャー
2018.04.18鬱の友達と心を通わせるにはビル・バーナット
2018.03.23脳に良い変化をもたらす運動の効果ウェンディー・スズキ
おすすめ 12018.03.21物事の「良し悪し」は思い込みに過ぎないヘザー・ラニエ
おすすめ 12018.01.19脳が深い睡眠から更なる恩恵を得る方法ダン・ガーテンバーグ
2018.01.04うつを一人で抱え込まないでニッキー・ウェバー・アレン
2017.10.26長寿の秘訣は周囲の人との交流かもスーザン・ピンカー
2017.09.04脳はどのように美しさを判定するか?アンジャン・チャタジー
2017.08.22脳が「意識された現実」という幻覚を作り出す仕組みアニル・セス
2017.07.18注意を向けた時、脳では何が起きているのかメディ・オディカニ=セイドラー
2017.07.12ティーンエイジャーの登校時間を遅らせるべき理由とはウェンディ・トロクセル
2017.06.09死に直面したとき、人生に生きる価値を与えてくれるのはルーシー・カラニシ
おすすめ 12017.06.07最善の自己と最悪の自己の生物学ロバート・サポルスキー
2017.05.31メンタルヘルスを気遣うのは恥ずかしくなんかないサング・デリ
2017.05.26
洋楽 おすすめ
RECOMMENDS
洋楽歌詞
ステイザ・キッド・ラロイ、ジャスティン・ビーバー
洋楽最新ヒット2021.08.20スピーチレス~心の声ナオミ・スコット
洋楽最新ヒット2019.05.23シェイプ・オブ・ユーエド・シーラン
洋楽人気動画2017.01.30フェイデッドアラン・ウォーカー
洋楽人気動画2015.12.03ウェイティング・フォー・ラヴアヴィーチー
洋楽人気動画2015.06.26シー・ユー・アゲインウィズ・カリファ
洋楽人気動画2015.04.06シュガーマルーン5
洋楽人気動画2015.01.14シェイク・イット・オフテイラー・スウィフト
ポップス2014.08.18オール・アバウト・ザット・ベースメーガン・トレイナー
ポップス2014.06.11ストーリー・オブ・マイ・ライフワン・ダイレクション
洋楽人気動画2013.11.03コール・ミー・メイビーカーリー・レイ・ジェプセン
洋楽人気動画2012.03.01美しき生命コールドプレイ
洋楽人気動画2008.08.04バッド・デイ~ついてない日の応援歌ダニエル・パウター
洋楽人気動画2008.05.14サウザンド・マイルズヴァネッサ・カールトン
洋楽人気動画2008.02.19イッツ・マイ・ライフボン・ジョヴィ
ロック2007.10.11アイ・ウォント・イット・ザット・ウェイバックストリート・ボーイズ
洋楽人気動画2007.09.14マイ・ハート・ウィル・ゴー・オンセリーヌ・ディオン
洋楽人気動画2007.07.12ヒーローマライア・キャリー
洋楽人気動画2007.03.21オールウェイズ・ラヴ・ユーホイットニー・ヒューストン
洋楽人気動画2007.02.19オネスティビリー・ジョエル
洋楽人気動画2005.09.16