
TED日本語
TED Talks(英語 日本語字幕付き動画)
TED日本語 - ハリー・クリフ: 物理学は終焉に達したのか?
TED Talks
物理学は終焉に達したのか?
Have we reached the end of physics?
ハリー・クリフ
Harry Cliff
内容
なぜ物は存在しているのでしょう?宇宙に面白いものがこうもたくさんあるのはどうしてなのでしょう?CERNの大型ハドロン衝突型加速器を使って研究する素粒子物理学者であるハリー・クリフのメッセージは、そのような疑問への答えを求める人たちにとって悪い知らせかもしれません。地上最大の装置と科学者達の最善の努力にもかかわらず、自然の持つ奇妙な性質を説明しきることは出来ないかもしれないのです。これは物理学の終焉を意味するのでしょうか?宇宙が秘める構造の研究状況を伝えるこの刺激的な講演に耳を傾けましょう。
字幕
SCRIPT
Script
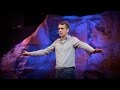
A hundred years ago this month, a 36-year-old Albert Einstein stood up in front of the Prussian Academy of Sciences in Berlin to present a radical new theory of space, time and gravity: the general theory of relativity.
General relativity is unquestionably Einstein's masterpiece, a theory which reveals the workings of the universe at the grandest scales, capturing in one beautiful line of algebra everything from why apples fall from trees to the beginning of time and space.
1915 must have been an exciting year to be a physicist. Two new ideas were turning the subject on its head. One was Einstein's theory of relativity, the other was arguably even more revolutionary: quantum mechanics, a mind-meltingly strange yet stunningly successful new way of understanding the microworld, the world of atoms and particles.
Over the last century, these two ideas have utterly transformed our understanding of the universe. It's thanks to relativity and quantum mechanics that we've learned what the universe is made from, how it began and how it continues to evolve. A hundred years on, we now find ourselves at another turning point in physics, but what's at stake now is rather different. The next few years may tell us whether we'll be able to continue to increase our understanding of nature, or whether maybe for the first time in the history of science, we could be facing questions that we can not answer, not because we don't have the brains or technology, but because the laws of physics themselves forbid it.
This is the essential problem: the universe is far, far too interesting. Relativity and quantum mechanics appear to suggest that the universe should be a boring place. It should be dark, lethal and lifeless. But when we look around us, we see we live in a universe full of interesting stuff, full of stars, planets, trees, squirrels. The question is, ultimately, why does all this interesting stuff exist? Why is there something rather than nothing? This contradiction is the most pressing problem in fundamental physics, and in the next few years, we may find out whether we'll ever be able to solve it.
At the heart of this problem are two numbers,two extremely dangerous numbers. These are properties of the universe that we can measure, and they're extremely dangerous because if they were different, even by a tiny bit, then the universe as we know it would not exist. The first of these numbers is associated with the discovery that was made a few kilometers from this hall, at CERN, home of this machine, the largest scientific device ever built by the human race, the Large Hadron Collider. The LHC whizzes subatomic particles around a 27-kilometer ring, getting them closer and closer to the speed of light before smashing them into each other inside gigantic particle detectors. On July 4,2012, physicists at CERN announced to the world that they'd spotted a new fundamental particle being created at the violent collisions at the LHC: the Higgs boson.
Now, if you followed the news at the time, you'll have seen a lot of physicists getting very excited indeed, and you'd be forgiven for thinking we get that way every time we discover a new particle. Well, that is kind of true, but the Higgs boson is particularly special. We all got so excited because finding the Higgs proves the existence of a cosmic energy field. Now, you may have trouble imagining an energy field, but we've all experienced one. If you've ever held a magnet close to a piece of metal and felt a force pulling across that gap, then you've felt the effect of a field. And the Higgs field is a little bit like a magnetic field, except it has a constant value everywhere. It's all around us right now. We can't see it or touch it, but if it wasn't there, we would not exist. The Higgs field gives mass to the fundamental particles that we're made from. If it wasn't there, those particles would have no mass, and no atoms could form and there would be no us.
But there is something deeply mysterious about the Higgs field. Relativity and quantum mechanics tell us that it has two natural settings, a bit like a light switch. It should either be off, so that it has a zero value everywhere in space, or it should be on so it has an absolutely enormous value. In both of these scenarios, atoms could not exist, and therefore all the other interesting stuff that we see around us in the universe would not exist. In reality, the Higgs field is just slightly on, not zero but 10,000 trillion times weaker than its fully on value, a bit like a light switch that's got stuck just before the off position. And this value is crucial. If it were a tiny bit different, then there would be no physical structure in the universe.
So this is the first of our dangerous numbers, the strength of the Higgs field. Theorists have spent decades trying to understand why it has this very peculiarly fine-tuned number, and they've come up with a number of possible explanations. They have sexy-sounding names like "supersymmetry" or "large extra dimensions." I'm not going to go into the details of these ideas now, but the key point is this: if any of them explained this weirdly fine-tuned value of the Higgs field, then we should see new particles being created at the LHC along with the Higgs boson. So far, though, we've not seen any sign of them.
But there's actually an even worse example of this kind of fine-tuning of a dangerous number, and this time it comes from the other end of the scale, from studying the universe at vast distances. One of the most important consequences of Einstein's general theory of relativity was the discovery that the universe began as a rapid expansion of space and time 13.8 billion years ago, the Big Bang. Now, according to early versions of the Big Bang theory, the universe has been expanding ever since with gravity gradually putting the brakes on that expansion. But in 1998, astronomers made the stunning discovery that the expansion of the universe is actually speeding up. The universe is getting bigger and bigger faster and faster driven by a mysterious repulsive force called dark energy.
Now, whenever you hear the word "dark" in physics, you should get very suspicious because it probably means we don't know what we're talking about.
(Laughter)
We don't know what dark energy is, but the best idea is that it's the energy of empty space itself, the energy of the vacuum. Now, if you use good old quantum mechanics to work out how strong dark energy should be, you get an absolutely astonishing result. You find that dark energy should be 10 to the power of 120 times stronger than the value we observe from astronomy. That's one with 120 zeroes after it. This is a number so mind-bogglingly huge that it's impossible to get your head around. We often use the word "astronomical" when we're talking about big numbers. Well, even that one won't do here. This number is bigger than any number in astronomy. It's a thousand trillion trillion trillion times bigger than the number of atoms in the entire universe.
So that's a pretty bad prediction. In fact, it's been called the worst prediction in physics, and this is more than just a theoretical curiosity. If dark energy were anywhere near this strong, then the universe would have been torn apart, stars and galaxies could not form, and we would not be here. So this is the second of those dangerous numbers, the strength of dark energy, and explaining it requires an even more fantastic level of fine-tuning than we saw for the Higgs field. But unlike the Higgs field, this number has no known explanation.
The hope was that a complete combination of Einstein's general theory of relativity, which is the theory of the universe at grand scales, with quantum mechanics, the theory of the universe at small scales, might provide a solution. Einstein himself spent most of his later years on a futile search for a unified theory of physics, and physicists have kept at it ever since.
One of the most promising candidates for a unified theory is string theory, and the essential idea is, if you could zoom in on the fundamental particles that make up our world, you'd see actually that they're not particles at all, but tiny vibrating strings of energy, with each frequency of vibration corresponding to a different particle, a bit like musical notes on a guitar string.
So it's a rather elegant, almost poetic way of looking at the world, but it has one catastrophic problem. It turns out that string theory isn't one theory at all, but a whole collection of theories. It's been estimated, in fact, that there are 10 to the 500 different versions of string theory. Each one would describe a different universe with different laws of physics. Now, critics say this makes string theory unscientific. You can't disprove the theory. But others actually turned this on its head and said, well, maybe this apparent failure is string theory's greatest triumph. What if all of these 10 to the 500 different possible universes actually exist out there somewhere in some grand multiverse? Suddenly we can understand the weirdly fine-tuned values of these two dangerous numbers. In most of the multiverse, dark energy is so strong that the universe gets torn apart, or the Higgs field is so weak that no atoms can form. We live in one of the places in the multiverse where the two numbers are just right. We live in a Goldilocks universe.
Now, this idea is extremely controversial, and it's easy to see why. If we follow this line of thinking, then we will never be able to answer the question, "Why is there something rather than nothing?" In most of the multiverse, there is nothing, and we live in one of the few places where the laws of physics allow there to be something. Even worse, we can't test the idea of the multiverse. We can't access these other universes, so there's no way of knowing whether they're there or not.
So we're in an extremely frustrating position. That doesn't mean the multiverse doesn't exist. There are other planets, other stars, other galaxies, so why not other universes? The problem is, it's unlikely we'll ever know for sure. Now, the idea of the multiverse has been around for a while, but in the last few years, we've started to get the first solid hints that this line of reasoning may get born out. Despite high hopes for the first run of the LHC, what we were looking for there -- we were looking for new theories of physics: supersymmetry or large extra dimensions that could explain this weirdly fine-tuned value of the Higgs field. But despite high hopes, the LHC revealed a barren subatomic wilderness populated only by a lonely Higgs boson. My experiment published paper after paper where we glumly had to conclude that we saw no signs of new physics.
The stakes now could not be higher. This summer, the LHC began its second phase of operation with an energy almost double what we achieved in the first run. What particle physicists are all desperately hoping for are signs of new particles, micro black holes, or maybe something totally unexpected emerging from the violent collisions at the Large Hadron Collider. If so, then we can continue this long journey that began 100 years ago with Albert Einstein towards an ever deeper understanding of the laws of nature.
But if, in two or three years' time, when the LHC switches off again for a second long shutdown, we've found nothing but the Higgs boson, then we may be entering a new era in physics: an era where there are weird features of the universe that we can not explain; an era where we have hints that we live in a multiverse that lies frustratingly forever beyond our reach; an era where we will never be able to answer the question, "Why is there something rather than nothing?"
Thank you.
(Applause)
Bruno Giussani: Harry, even if you just said the science may not have some answers, I would like to ask you a couple of questions, and the first is: building something like the LHC is a generational project. I just mentioned, introducing you, that we live in a short-term world. How do you think so long term, projecting yourself out a generation when building something like this?
Harry Cliff: I was very lucky that I joined the experiment I work on at the LHC in 2008, just as we were switching on, and there are people in my research group who have been working on it for three decades, their entire careers on one machine. So I think the first conversations about the LHC were in 1976, and you start planning the machine without the technology that you know you're going to need to be able to build it. So the computing power did not exist in the early '90s when design work began in earnest. One of the big detectors which record these collisions, they didn't think there was technology that could withstand the radiation that would be created in the LHC, so there was basically a lump of lead in the middle of this object with some detectors around the outside, but subsequently we have developed technology. So you have to rely on people's ingenuity, that they will solve the problems, but it may be a decade or more down the line.
BG: China just announced two or three weeks ago that they intend to build a supercollider twice the size of the LHC. I was wondering how you and your colleagues welcome the news.
HC: Size isn't everything, Bruno. BG: I'm sure. I'm sure.
(Laughter)
It sounds funny for a particle physicist to say that. But I mean, seriously, it's great news. So building a machine like the LHC requires countries from all over the world to pool their resources. No one nation can afford to build a machine this large, apart from maybe China, because they can mobilize huge amounts of resources, manpower and money to build machines like this. So it's only a good thing. What they're really planning to do is to build a machine that will study the Higgs boson in detail and could give us some clues as to whether these new ideas, like supersymmetry, are really out there, so it's great news for physics, I think.
BG: Harry, thank you. HC: Thank you very much.
(Applause)
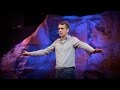
100年前の今月 36歳のアルベルト・アインシュタインは ベルリンにある プロイセン科学アカデミーで 空間と時間と重力に関する 画期的な理論を発表しました 一般相対性理論です
一般相対性理論は疑いなく アインシュタインの最高傑作で 大きなスケールでの 宇宙の仕組みを明らかにし 1行の美しい方程式によって リンゴが木から落ちる理由から 時空間の始まりまで説明します
1915年は物理学者にとって エキサイティングな年だったに違いありません 2つの新しいアイデアが 世界の見方を一新しました 1つは アインシュタインの 相対性理論で もう1つは さらに革命的とも言える 量子力学です 頭がおかしくなりそうなくらい 奇妙でありながら 原子や素粒子のなすミクロの世界が 驚くほどうまく説明できます
この1世紀の間に この2つのアイデアは 宇宙に関する私たちの理解を すっかり変えました 宇宙が何でできていて どのように始まり どう進化しているのか 私たちが知っているのも 相対性理論と 量子力学のお陰です 100年経った今 私たちは物理学における 別の転換点に差し掛かっていますが 様相が異なっています この先数年で結果が 出るかもしれません 果たして私たちは自然についての理解を 広げ続けていくことができるのか それとも科学の歴史で初めて 答えることの出来ない問に 直面することになるのか 知恵や技術が 足りないからではなく 物理法則がそれを 認めないためにです
問題の要点は 宇宙が面白すぎるということです 相対性理論と 量子力学によれば 宇宙はもっと退屈な場所で あるはずなんです 暗く 命を寄せ付けない 死んだ世界です しかし周りを見渡してみると 私たちが生きている宇宙は面白いものに溢れています 満天の星々 惑星 木々 リス 疑問は なぜこんな面白いものが 存在しているのか ということです なぜ無ではなく 物の存在があるのか? この矛盾は 基礎物理学において 最も差し迫った問題で 私たちに この問題を解くことができるのか 今後数年で 答えが出るかもしれません
この問題の中心には 2つの数値があります とても危険な数値です それは宇宙の性質を示す 測定可能な値で 危険だというのは それがほんのわずかでも 違っていたなら 我々の知る宇宙は 存在しないからです 2つの数値の1つは この会場からすぐ近くにある CERNで発見されたことに 関係しています CERNには この 人類が作り上げた 最大の科学装置があります 大型ハドロン衝突型加速器(LHC)です この装置は 全周約27キロの輪の中で 素粒子を 光速に近いスピードまで加速し 巨大な粒子検知器の中で ぶつかり合わせます 2012年7月4日 CERNの物理学者が LHCによる猛烈な衝突により 新たな基本粒子が生成されたと発表しました ヒッグス粒子です
当時ニュースを チェックしていた人は 多くの物理学者がとても興奮していたのを 見たことでしょう 新しい粒子が見つかるたびに 物理学者は あんなバカ騒ぎをするのかと 思ったかもしれません そういう面もありますが ビッグス粒子は ちょっと特別なんです 私たちが興奮したのは ビッグス粒子の発見は 普遍的エネルギー場の存在を 証明するからです エネルギー場と言われても 分かりにくいかもしれませんが 誰もが体験している ものがあります 磁石を鉄に近づけると 間を隔てて引き合う力を 感じるはずですが それは場の効果を 感じているんです ヒッグス場は 磁場に似ていますが 違うのは 値がどこでも一定だということです 今も私たちの周りに 存在しています 見ることも 触れることも できませんが もしそれがなかったなら 私たちは存在していないのです ヒッグス場は 私たちを形作る基本粒子に 質量を与えます それがなければ 粒子は質量を持たず 原子が形成されることもなく 我々も存在しないのです
しかしヒッグス場には ひどく不思議なところがあります 相対性理論と量子力学は それには電気のスイッチのように 自然な2つの状態があることを 示しています オフ状態で 宇宙のどこでもゼロか オン状態で 膨大な値を持つかです どちらの場合でも 原子は存在できず 私たちが宇宙で目にする あらゆる興味深いものもまた 存在しません 実際には ヒッグス場は かすかにオンの状態で ゼロではありませんが 完全にオンの状態より1京倍弱く 電気スイッチがオフの手前で 引っかかっているような状態です この値はとても重要です わずかでも違っていたら 宇宙に物理的構造が 存在しないからです
これが危険な値の1つ目 ヒッグス場の強さです 理論物理学者は なぜこのような 妙な値になっているのか知ろうと 何十年も努力を続け 様々な説明を考え出し 「超対称性」とか 「大きな余剰次元」みたいな かっこいい名前をつけています ここで立ち入った 説明はしませんが 鍵になるのは これらのどれにせよ ヒッグス場の奇妙な値を説明できるなら LHCでヒッグス粒子とともに 生成される新たな粒子が 観測されるはずだ ということです これまでのところ そのようなものの兆候は 見つかっていません
実は こういう危険な値が 妙な値をしているという さらにまずい例があって それはスケール的に反対の極端 遙か彼方の宇宙の 研究から来ています アインシュタインの一般相対性理論の 最も重要な帰結の1つは 宇宙がビッグバンと呼ばれる 138億年前の急激な時空の膨張で 始まったと分かったことです 初期のビッグバン理論では 宇宙の膨張は 重力の力によって 徐々に遅くなっていくと 考えられていました しかし1998年に天文学者が 驚くべきことを発見しました 宇宙の膨張は 加速しているのです 宇宙はますます速く 大きくなっていて それを後押ししているのが 謎の反発力 ダークエネルギーです
物理学で「ダーク」という 言葉を聞いたときは 疑ってかかってください 物理学者はそれが何か よく分かってないことを意味するからです
(笑)
ダークエネルギーが 何なのか分かりませんが あえて言うなら 何もない空間の持つエネルギー 真空のエネルギーです 古典的な量子力学を使って ダークエネルギーの 強さを計算すると まったく驚くような 結果になります ダークエネルギーは 天文学で観察される値よりも 10の120乗倍 強いはずなのです 1の後に0が 120個付く ということです これはまったく 目が回るような値で 理解不能です 大きな数字のことをよく 「天文学的」と言いますが それでさえ不足です この値は天文学における いかなる値よりも大きく 千の 1兆倍の 1兆倍の 1兆倍 宇宙にある原子の総数より 大きいのです
極めてまずい予測です 実際これは物理学における 最悪の予測だと言われてきました しかもこれは理論的な 興味だけの話ではありません もしダークエネルギーが それほど強いのだとしたら 宇宙はバラバラになり 銀河は形成されず 我々もここに存在しません これが危険な数字の2つ目 タークエネルギーの強さです これを説明するためには ヒッグス場よりもさらに曲芸的な 調整が必要になります ヒッグス場とは異なり この値を説明できるものは知られていません
希望が持たれていたのは 大きなスケールの 宇宙の理論である アインシュタインの 相対性理論と 小さなスケールの宇宙の理論である 量子力学の完璧な組み合わせによって 解決できるかもしれない ということでした アインシュタイン自身 後年は物理学の統一理論を求める 実りのない探求に多くの時間を費やし それ以来 多くの物理学者たちが 取り組んできました
統一理論の候補として 最も有望視されているのは弦理論で その基本的なアイデアは 世界を構成する基本粒子を 拡大していくと それは粒子ではなく 小さな振動する エネルギーの弦で 振動周波数ごとに 異なる粒子に対応していて ギターの弦に対する音符のようなものだ ということです
これはエレガントで ほとんど詩的とも言える世界の見方ですが 実は 救いがたい 問題があります 弦理論というのは 1つの理論ではなく 大きな理論の集まりなのです 弦理論には異なるバージョンが 10の500乗個あると 見積もられています そのそれぞれが 異なる物理法則を持った 異なる宇宙を記述しているのです そんなの科学とは言えない という批判があります この理論は反証し得ないと 一方で 弦理論の破綻と見えることが 実は最大の利点かもしれないと 見る人々もいます その10の500乗個の 異なる可能な宇宙が 実際 多元宇宙のどこかに 存在しているとしたら? そうすると突然 あの2つの危険な数値が 奇妙な値をしていることも 理解できるようになります 多元宇宙のほとんどでは ダークエネルギーが強すぎて 宇宙がバラバラになってしまうか ヒッグス場が弱すぎて 原子が形成されないが 私たちは たまたま 2つの値が適切な値をしている場所に 生きているということです ゴルディロックスの宇宙です
このアイデアには極めて議論が 多いのも分かるでしょう このような考え方では 「無ではなく物が存在するのはなぜか」 という問に 決して答えることができません 多元宇宙の多くには何もなく 物理法則が物の存在を許容する わずかな宇宙の1つに 我々が住んでいる というだけの話です さらにまずいのは この多元宇宙のアイデアは 確認できないということです 他の宇宙を観測する方法はなく それが存在するのかしないのか 知りようがないのです
我々は極めて 苛立たしい状況にあります 多元宇宙など存在しない というのではありません 他にも惑星があり 恒星があり 銀河があるのだから 他の宇宙があっても おかしくはないでしょう 問題は 我々が確証を得ることは ないだろうということです 多元宇宙のアイデアが出て来て 結構経ちますが この数年で このような理論が 裏付けられるかもしれない 最初の兆しが 得られ始めました LHCが最初に稼働した時以来 私たちが大きな期待を よせていたのは 物理の新しい理論の発見です 超対称性であれ 大きな余剰次元であれ ヒッグス場の奇妙な値を 説明できる何かです しかし大きな期待をよそに LHCが見せてきたのは ヒッグス粒子1つだけという 不毛な荒野です 実験から生まれた 数々の論文はどれも 新しい物理学の兆候は見られなかったと 辛気くさく締めくくらざるを得ませんでした
今や掛け金は これ以上なく高くなっています この夏 LHCは 第2期の稼働を始め 第1期のほとんど倍のエネルギーで 動かしています 素粒子物理学者はみんな 新しい粒子であれ マイクロ・ブラックホールであれ あるいはまったく 予期しないものであれ LHCでの猛烈な衝突で何らかの兆候が 見つかるのを切に願っています そうなれば 私たちは アインシュタインが 100年前に始めた長い旅を 自然法則のより深い理解に向けて 続けていくことができます
しかし2、3年稼働したのち LHCが再び長い休止期間に入るとき 我々に見つかったものが ヒッグス粒子だけだったとしたら 物理学は 新たな時代に 入るかもしれません それは我々に説明し得ない 奇妙な性質が宇宙にある時代 永遠に手の届かない 多元宇宙の中に 我々は生きているらしい 気配のある時代 「無ではなく物の存在があるのはなぜか」 という問に 決して答えることの できない時代です
ありがとうございました
(拍手)
(ブルーノ・ジュッサーニ)ハリー 科学にも答えられないことが あるかもしれないとのことですが 2つお聞きしたいことがあります まず LHCのようなものを構築するというのは 長い期間を要するプロジェクトです あなたを紹介するとき 私たちは短期的な世界に 生きていると言いましたが あなたにはどうして こんなものを構築しようなどという 長期的な考え方ができるんですか?
(ハリー・クリフ)私はとても幸運でした 私がLHCを使う実験に 参加したのは2008年 ちょうど稼働を始めた時です 私たちの研究グループの中には これに30年も取り組んできた人たちもいて 1台の装置に 生涯をかけています LHCについて最初の議論が行われたのは 1976年のことですが その構築に必要だと分かっている技術が まだ存在しない段階で 計画を始めていたわけです 本格的に設計が始まった 90年代初期には 必要とされる計算能力が ありませんでした 衝突を記録する 大きな検出器がありますが LHCで生成される放射能に 耐えるような技術は ないと考えられていました それで基本的には 中心に鉛の塊があって 周りに検出器が あるような感じですが 追々技術を 開発していったんです 人々が発明の才で問題を解決してくれるのを 期待して進めるしかなく それには何十年も かかるかもしれません
(ジュッサーニ)中国が 2、3週間前に LHCより2倍大きい 超衝突型加速器の 建設計画を 発表しました あなたや同僚の人たちは このニュースをどう受け止めましたか?
(クリフ)大きさがすべてじゃないさ (ジュッサーニ)そりゃそうですとも
(笑)
素粒子物理学者が 言うのも変ですが まじめな話 素晴らしいニュースだと思います LHCのような装置を 建設するには 世界中の国が リソースを結集する必要があります これほど大きな機械を 一国で作ることはできませんが 中国は例外かもしれません このような機械の 建設のために 膨大な人・金・資源を 動員できますから これはもちろん 悪い話ではありません 彼らが計画しているのは その機械によって ヒッグス粒子について詳細に研究し 超対称性のような新しいアイデアが 本物なのか手がかりを得るということで 物理学にとって 素晴らしいニュースだと思います
(ジュッサーニ)ハリー どうもありがとう (クリフ)こちらこそ
(拍手)
品詞分類
- 主語
- 動詞
- 助動詞
- 準動詞
- 関係詞等
TED 日本語
TED Talks
関連動画
謎に包まれた土星の衛星タイタンを調べて生命の起源に関して何がわかるかエリザベス・ジビ・タートル
2020.09.17宇宙に関する理解に疑問を抱かせる珍しい銀河ブーチン・ムトゥル・パクディル
2018.09.18宇宙人はどこにいるのでしょう?スティーヴン・ウェッブ
2018.08.16他の恒星系からの初の訪問者オウムアムアカレン・J・ミーチ
2018.07.19地球上で最も火星によく似た場所アルマンド・アズア・ブストス
2017.10.05あなたは皆既日食を是非体験すべきであるデイヴィッド・バロン
2017.08.10ブラックホールの写真を撮影するケイティ・バウマン
2017.04.28地球を周回している宇宙ゴミを片付けましょうナタリー・パネク
2017.01.05地球外生命を宿しているかもしれない1つの惑星と3つの衛星ジェームズ・グリーン
2016.09.07惑星が生命を育むために必要なものデイヴ・ブレイン
2016.09.04火星に移住する子供達が生き抜く方法スティーブン・ペトラネック
2016.05.05宇宙でもっとも神秘的な星タベサ・ボヤジアン
2016.04.29重力波発見が意味することアラン・アダムス
2016.03.10他の惑星の生命を見つけ出す方法アオマワ・シールズ
2016.01.28火星は予備の地球ではないルシアン・ウォーコウィッチ
2016.01.14宇宙の誕生が見える最新型望遠鏡ウェンディ・フリードマン
2015.09.22
洋楽 おすすめ
RECOMMENDS
洋楽歌詞
ダイナマイトビーティーエス
洋楽最新ヒット2020.08.20ディス・イズ・ミーグレイテスト・ショーマン・キャスト
洋楽人気動画2018.01.11グッド・ライフGイージー、ケラーニ
洋楽人気動画2017.01.27ホワット・ドゥ・ユー・ミーン?ジャスティン・ビーバー
洋楽人気動画2015.08.28ファイト・ソングレイチェル・プラッテン
洋楽人気動画2015.05.19ラヴ・ミー・ライク・ユー・ドゥエリー・ゴールディング
洋楽人気動画2015.01.22アップタウン・ファンクブルーノ・マーズ、マーク・ロンソン
洋楽人気動画2014.11.20ブレイク・フリーアリアナ・グランデ
洋楽人気動画2014.08.12ハッピーファレル・ウィリアムス
ポップス2014.01.08カウンティング・スターズワンリパブリック
ロック2013.05.31ア・サウザンド・イヤーズクリスティーナ・ペリー
洋楽人気動画2011.10.26ユー・レイズ・ミー・アップケルティック・ウーマン
洋楽人気動画2008.05.30ルーズ・ユアセルフエミネム
洋楽人気動画2008.02.21ドント・ノー・ホワイノラ・ジョーンズ
洋楽人気動画2008.02.15オンリー・タイムエンヤ
洋楽人気動画2007.10.03ミス・ア・シングエアロスミス
ロック2007.08.18タイム・トゥ・セイ・グッバイサラ・ブライトマン
洋楽人気動画2007.06.08シェイプ・オブ・マイ・ハートスティング
洋楽人気動画2007.03.18ウィ・アー・ザ・ワールド(U.S.A. フォー・アフリカ)マイケル・ジャクソン
洋楽人気動画2006.05.14ホテル・カリフォルニアイーグルス
ロック2005.07.06