
TED日本語
TED Talks(英語 日本語字幕付き動画)
TED日本語 - アンドレア・ゲッズ: 超大質量ブラックホールを探す
TED Talks
超大質量ブラックホールを探す
The hunt for a supermassive black hole
アンドレア・ゲッズ
Andrea Ghez
内容
ケック望遠校の最新データを元に、アンドレア・ゲッズは、宇宙最大の謎であるブラックホールの理解を、最高水準の補償光学がいかに助けているかを示します。彼女は、天の川銀河の中心に超大質量ブラックホールが存在する証拠について話します。
字幕
SCRIPT
Script
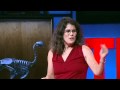
How do you observe something you can't see? This is the basic question of somebody who's interested in finding and studying black holes. Because black holes are objects whose pull of gravity is so intense that nothing can escape it, not even light, so you can't see it directly.
So, my story today about black holes is about one particular black hole. I'm interested in finding whether or not there is a really massive, what we like to call "supermassive" black hole at the center of our galaxy. And the reason this is interesting is that it gives us an opportunity to prove whether or not these exotic objects really exist. And second, it gives us the opportunity to understand how these supermassive black holes interact with their environment, and to understand how they affect the formation and evolution of the galaxies which they reside in.
So, to begin with, we need to understand what a black hole is so we can understand the proof of a black hole. So, what is a black hole? Well, in many ways a black hole is an incredibly simple object, because there are only three characteristics that you can describe: the mass, the spin, and the charge. And I'm going to only talk about the mass. So, in that sense, it's a very simple object. But in another sense, it's an incredibly complicated object that we need relatively exotic physics to describe, and in some sense represents the breakdown of our physical understanding of the universe.
But today, the way I want you to understand a black hole, for the proof of a black hole, is to think of it as an object whose mass is confined to zero volume. So, despite the fact that I'm going to talk to you about an object that's supermassive, and I'm going to get to what that really means in a moment, it has no finite size. So, this is a little tricky.
But fortunately there is a finite size that you can see, and that's known as the Schwarzschild radius. And that's named after the guy who recognized why it was such an important radius. This is a virtual radius, not reality; the black hole has no size. So why is it so important? It's important because it tells us that any object can become a black hole. That means you, your neighbor, your cellphone, the auditorium can become a black hole if you can figure out how to compress it down to the size of the Schwarzschild radius.
At that point, what's going to happen? At that point gravity wins. Gravity wins over all other known forces. And the object is forced to continue to collapse to an infinitely small object. And then it's a black hole. So, if I were to compress the Earth down to the size of a sugar cube, it would become a black hole, because the size of a sugar cube is its Schwarzschild radius.
Now, the key here is to figure out what that Schwarzschild radius is. And it turns out that it's actually pretty simple to figure out. It depends only on the mass of the object. Bigger objects have bigger Schwarzschild radii. Smaller objects have smaller Schwarzschild radii. So, if I were to take the sun and compress it down to the scale of the University of Oxford, it would become a black hole.
So, now we know what a Schwarzschild radius is. And it's actually quite a useful concept, because it tells us not only when a black hole will form, but it also gives us the key elements for the proof of a black hole. I only need two things. I need to understand the mass of the object I'm claiming is a black hole, and what its Schwarzschild radius is. And since the mass determines the Schwarzschild radius, there is actually only one thing I really need to know.
So, my job in convincing you that there is a black hole is to show that there is some object that's confined to within its Schwarzschild radius. And your job today is to be skeptical. Okay, so, I'm going to talk about no ordinary black hole; I'm going to talk about supermassive black holes.
So, I wanted to say a few words about what an ordinary black hole is, as if there could be such a thing as an ordinary black hole. An ordinary black hole is thought to be the end state of a really massive star's life. So, if a star starts its life off with much more mass than the mass of the Sun, it's going to end its life by exploding and leaving behind these beautiful supernova remnants that we see here. And inside that supernova remnant is going to be a little black hole that has a mass roughly three times the mass of the Sun. On an astronomical scale that's a very small black hole.
Now, what I want to talk about are the supermassive black holes. And the supermassive black holes are thought to reside at the center of galaxies. And this beautiful picture taken with the Hubble Space Telescope shows you that galaxies come in all shapes and sizes. There are big ones. There are little ones. Almost every object in that picture there is a galaxy. And there is a very nice spiral up in the upper left. And there are a hundred billion stars in that galaxy, just to give you a sense of scale. And all the light that we see from a typical galaxy, which is the kind of galaxies that we're seeing here, comes from the light from the stars. So, we see the galaxy because of the star light.
Now, there are a few relatively exotic galaxies. I like to call these the prima donna of the galaxy world, because they are kind of show offs. And we call them active galactic nuclei. And we call them that because their nucleus, or their center, are very active. So, at the center there, that's actually where most of the starlight comes out from. And yet, what we actually see is light that can't be explained by the starlight. It's way more energetic. In fact, in a few examples it's like the ones that we're seeing here. There are also jets emanating out from the center. Again, a source of energy that's very difficult to explain if you just think that galaxies are composed of stars.
So, what people have thought is that perhaps there are supermassive black holes which matter is falling on to. So, you can't see the black hole itself, but you can convert the gravitational energy of the black hole into the light we see. So, there is the thought that maybe supermassive black holes exist at the center of galaxies. But it's a kind of indirect argument.
Nonetheless, it's given rise to the notion that maybe it's not just these prima donnas that have these supermassive black holes, but rather all galaxies might harbor these supermassive black holes at their centers. And if that's the case -- and this is an example of a normal galaxy; what we see is the star light. And if there is a supermassive black hole, what we need to assume is that it's a black hole on a diet. Because that is the way to suppress the energetic phenomena that we see in active galactic nuclei.
If we're going to look for these stealth black holes at the center of galaxies, the best place to look is in our own galaxy, our Milky Way. And this is a wide field picture taken of the center of the Milky Way. And what we see is a line of stars. And that is because we live in a galaxy which has a flattened, disk-like structure. And we live in the middle of it, so when we look towards the center, we see this plane which defines the plane of the galaxy, or line that defines the plane of the galaxy.
Now, the advantage of studying our own galaxy is it's simply the closest example of the center of a galaxy that we're ever going to have, because the next closest galaxy is 100 times further away. So, we can see far more detail in our galaxy than anyplace else. And as you'll see in a moment, the ability to see detail is key to this experiment.
So, how do astronomers prove that there is a lot of mass inside a small volume? Which is the job that I have to show you today. And the tool that we use is to watch the way stars orbit the black hole. Stars will orbit the black hole in the very same way that planets orbit the sun. It's the gravitational pull that makes these things orbit. If there were no massive objects these things would go flying off, or at least go at a much slower rate because all that determines how they go around is how much mass is inside its orbit.
So, this is great, because remember my job is to show there is a lot of mass inside a small volume. So, if I know how fast it goes around, I know the mass. And if I know the scale of the orbit I know the radius. So, I want to see the stars that are as close to the center of the galaxy as possible. Because I want to show there is a mass inside as small a region as possible. So, this means that I want to see a lot of detail. And that's the reason that for this experiment we've used the world's largest telescope.
This is the Keck observatory. It hosts two telescopes with a mirror 10 meters, which is roughly the diameter of a tennis court. Now, this is wonderful, because the campaign promise of large telescopes is that is that the bigger the telescope, the smaller the detail that we can see. But it turns out these telescopes, or any telescope on the ground has had a little bit of a challenge living up to this campaign promise. And that is because of the atmosphere. Atmosphere is great for us; it allows us to survive here on Earth. But it's relatively challenging for astronomers who want to look through the atmosphere to astronomical sources.
So, to give you a sense of what this is like, it's actually like looking at a pebble at the bottom of a stream. Looking at the pebble on the bottom of the stream, the stream is continuously moving and turbulent, and that makes it very difficult to see the pebble on the bottom of the stream. Very much in the same way, it's very difficult to see astronomical sources, because of the atmosphere that's continuously moving by.
So, I've spent a lot of my career working on ways to correct for the atmosphere, to give us a cleaner view. And that buys us about a factor of 20. And I think all of you can agree that if you can figure out how to improve life by a factor of 20, you've probably improved your lifestyle by a lot, say your salary, you'd notice, or your kids, you'd notice.
And this animation here shows you one example of the techniques that we use, called adaptive optics. You're seeing an animation that goes between an example of what you would see if you don't use this technique -- in other words, just a picture that shows the stars -- and the box is centered on the center of the galaxy, where we think the black hole is. So, without this technology you can't see the stars. With this technology all of a sudden you can see it. This technology works by introducing a mirror into the telescope optics system that's continuously changing to counteract what the atmosphere is doing to you. So, it's kind of like very fancy eyeglasses for your telescope.
Now, in the next few slides I'm just going to focus on that little square there. So, we're only going to look at the stars inside that small square, although we've looked at all of them. So, I want to see how these things have moved. And over the course of this experiment, these stars have moved a tremendous amount. So, we've been doing this experiment for 15 years, and we see the stars go all the way around.
Now, most astronomers have a favorite star, and mine today is a star that's labeled up there, SO-2. Absolutely my favorite star in the world. And that's because it goes around in only 15 years. And to give you a sense of how short that is, the sun takes 200 million years to go around the center of the galaxy. Stars that we knew about before, that were as close to the center of the galaxy as possible, take 500 years. And this one, this one goes around in a human lifetime. That's kind of profound, in a way.
But it's the key to this experiment. The orbit tells me how much mass is inside a very small radius. So, next we see a picture here that shows you before this experiment the size to which we could confine the mass of the center of the galaxy. What we knew before is that there was four million times the mass of the sun inside that circle. And as you can see, there was a lot of other stuff inside that circle. You can see a lot of stars. So, there was actually lots of alternatives to the idea that there was a supermassive black hole at the center of the galaxy, because you could put a lot of stuff in there.
But with this experiment, we've confined that same mass to a much smaller volume that's 10,000 times smaller. And because of that, we've been able to show that there is a supermassive black hole there. To give you a sense of how small that size is, that's the size of our solar system. So, we're cramming four million times the mass of the sun into that small volume.
Now, truth in advertising. Right? I have told you my job is to get it down to the Schwarzchild radius. And the truth is, I'm not quite there. But we actually have no alternative today to explaining this concentration of mass. And, in fact, it's the best evidence we have to date for not only existence of a supermassive black hole at the center of our own galaxy, but any in our universe. So, what next? I actually think this is about as good as we're going to do with today's technology, so let's move on with the problem.
So, what I want to tell you, very briefly, is a few examples of the excitement of what we can do today at the center of the galaxy, now that we know that there is, or at least we believe, that there is a supermassive black hole there. And the fun phase of this experiment is, while we've tested some of our ideas about the consequences of a supermassive black hole being at the center of our galaxy, almost every single one has been inconsistent with what we actually see. And that's the fun.
So, let me give you the two examples. You can ask, "What do you expect for the old stars, stars that have been around the center of the galaxy for a long time, they've had plenty of time to interact with the black hole." What you expect there is that old stars should be very clustered around the black hole. You should see a lot of old stars next to that black hole.
Likewise, for the young stars, or in contrast, the young stars, they just should not be there. A black hole does not make a kind neighbor to a stellar nursery. To get a star to form, you need a big ball of gas and dust to collapse. And it's a very fragile entity. And what does the big black hole do? It strips that gas cloud apart. It pulls much stronger on one side than the other and the cloud is stripped apart. In fact, we anticipated that star formation shouldn't proceed in that environment.
So, you shouldn't see young stars. So, what do we see? Using observations that are not the ones I've shown you today, we can actually figure out which ones are old and which ones are young. The old ones are red. The young ones are blue. And the yellow ones, we don't know yet. So, you can already see the surprise. There is a dearth of old stars. There is an abundance of young stars, so it's the exact opposite of the prediction.
So, this is the fun part. And in fact, today, this is what we're trying to figure out, this mystery of how do you get -- how do you resolve this contradiction. So, in fact, my graduate students are, at this very moment, today, at the telescope, in Hawaii, making observations to get us hopefully to the next stage, where we can address this question of why are there so many young stars, and so few old stars. To make further progress we really need to look at the orbits of stars that are much further away. To do that we'll probably need much more sophisticated technology than we have today.
Because, in truth, while I said we're correcting for the Earth's atmosphere, we actually only correct for half the errors that are introduced. We do this by shooting a laser up into the atmosphere, and what we think we can do is if we shine a few more that we can correct the rest. So this is what we hope to do in the next few years. And on a much longer time scale, what we hope to do is build even larger telescopes, because, remember, bigger is better in astronomy.
So, we want to build a 30 meter telescope. And with this telescope we should be able to see stars that are even closer to the center of the galaxy. And we hope to be able to test some of Einstein's theories of general relativity, some ideas in cosmology about how galaxies form. So, we think the future of this experiment is quite exciting.
So, in conclusion, I'm going to show you an animation that basically shows you how these orbits have been moving, in three dimensions. And I hope, if nothing else, I've convinced you that,one, we do in fact have a supermassive black hole at the center of the galaxy. And this means that these things do exist in our universe, and we have to contend with this, we have to explain how you can get these objects in our physical world.
Second, we've been able to look at that interaction of how supermassive black holes interact, and understand, maybe, the role in which they play in shaping what galaxies are, and how they work.
And last but not least, none of this would have happened without the advent of the tremendous progress that's been made on the technology front. And we think that this is a field that is moving incredibly fast, and holds a lot in store for the future. Thanks very much. (Applause)
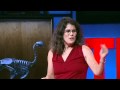
見えないものはどうやって観察できるでしょうか? ブラックホールを発見し、研究しようとする者にとって これは基本的な問いです なぜならブラックホールは その重力があまりに強力で 光でさえもそこから出てこられず つまり直接、目に見えないからです
今日私がお話するのは 特別なブラックホールについてです 私が興味を持っているのは 銀河の中心に、非常に大きい 「超大質量」のブラックホールがあるかどうかです なぜそれに興味があるかというと このような奇妙な物体が本当に 存在するかどうかを証明できる機会があるのと、 二つ目の理由は、それによって 超大質量ブラックホールが、どうやって 周辺環境と相互作用するか、また その周りにある銀河の形成と進化にどう影響するかを 理解する機会があるからです
まずはじめに ブラックホールの存在を信じられるよう ブラックホールについて理解しましょう ブラックホールとは何か? ある意味でそれは非常に単純な物体です なぜなら物理特性が三つしかないからです 質量と、 スピン(回転)と、電荷です 今日は質量についてだけお話します つまりある意味では非常に単純な物体ですが 別の意味では非常に複雑な物体で 奇妙な物理法則で記述しなくてはならず また、それが我々の宇宙に関す物理学的解釈の 崩壊を意味するからです
でも今日は ブラックホールを理解し それが実在することを理解していただくために その質量が体積ゼロの空間に 押し込められた物体を考えてみてください つまり超大質量を持つ 物体なのに 有限の大きさを持たないような物体がとはどんなものかを ご説明します ちょっとムズカシイわよね
でも、理解可能な有限の大きさがあり それは「シュヴァルツシルト半径」として知られています その大きさがいかに重要かを発見した 人の名前にちなみます これは仮想的な半径で、実在のものではありません ブラックホールには大きさがありませんから なぜ重要なのか? なぜならそれは、どんな物体でも ブラックホールになり得ることを示すからです つまりあなたや、隣人や、あなたの携帯電話や、 このホールなども、それを シュヴァルツシルト半径以下に押し縮めれば ブラックホールになるのです
そこまで行くとどうなるか? そこで重力が打ち勝ちます 他のすべての力に重力が打ち勝ち 物体は収縮を続け 無限に小さい物体になるのです それがブラックホールです 地球を角砂糖の大きさにまで押し縮めれば ブラックホールになります この場合、角砂糖の大きさがシュヴァルツシルト半径だからです
ここでの鍵は、シュヴァルツシルト半径は幾つか、ということです それを知るのはとても簡単だと分かりました それは物体の質量のみに依存します 大きい物体のシュヴァルツシルト半径は大きい 小さい物体では、小さい もし太陽を オックスフォード大学の大きさに押し縮めれば ブラックホールになるでしょう
シュヴァルツシルト半径はわかりましたね これはとても役に立つ概念で ブラックホールがいつできるかを 示すだけでなく ブラックホールの証明の鍵となる要素も与えてくれます 必要なのは二つだけ: ブラックホールだと証明したい 物体の質量と、 そのシュヴァルツシルト半径です そしてシュヴァルツシルト半径は質量で決まるので 実際知る必要があるのは質量だけです
つまり皆さんに、ブラックホールが 実在すると納得させるために そのシュヴァルツシルト半径内に収まる 物体が存在することを示すのが私の役割で 皆さんの役割は、疑い深くなることです それで、私は普通のブラックホールについては話しません 超大質量ブラックホールの話をします
そこでまず普通のブラックホールについて少し触れます 「普通の」ブラックホールがあるみたいにね 普通のブラックホールは、超巨星の生涯の 終末と考えられています つまり、太陽より遥かに巨大な質量をもって生まれた 恒星は、その生涯を終える時 爆発を起こし、 ご覧のような美しい超新星爆発の残骸を残します そしてその超新星爆発の残骸の中に 小さなブラックホールができます おおよそ太陽の質量の3倍くらいです 天文学的規模で言えば とても小さいブラックホールです
これからは超大質量ブラックホールについてお話します 超大質量ブラックホールは銀河の中心にあると考えられています ハッブル宇宙望遠鏡が撮影したこの美しい写真で 銀河があらゆる形をとることがわかります 大きいのもあるし、小さいのもあります この写真に見えるほとんど全てが銀河です その左上にとてもいい感じの渦巻状銀河があります その銀河には1千億個の恒星があります 大きさの感覚がわかりますか そしてちょうどご覧のこの銀河のような 銀河からやってくる全ての光は 恒星からやって来ます つまり恒星の光があるから銀河が見えます
中には非常に変わった銀河があります 「目立ちたがり」なので、私はそれを銀河世界の プリマンドンナと呼びます それは「活動銀河中心核」と呼ばれます なぜならその銀河中心核、あるいは 中央部は非常に活動的だからです つまりあの中心部分から ほとんどの星の光がやって来ます しかも恒星の光だけでは 説明できない光が見えているのです 予想よりずっとエネルギーが高い ちょうど私たちが見ているこういう銀河がです また、中央部からジェットが吹き出しています 銀河が恒星だけで出来ていると考えると このエネルギー源も説明がつきません
そこで超大質量ブラックホールがあり 物質がそこに落ち込んでいるのだろうと 推定されています つまりブラックホールを見ることはできないが そこの重力エネルギーが光に変換されて 見えているということです それが超大質量ブラックホールが銀河中央にあるかもしれないという 考え方が成立する理由です しかしそれは間接的な議論です
にもかかわらず、そこから 超大質量ブラックホールは プリマドンナ銀河だけでなく むしろ全ての銀河の中央に 存在するのではないかと考えられています もしそうなら―これは普通の銀河です 見えているのは恒星の光です もしここに超大質量ブラックホールがあるとするならば それはダイエット中だ、ということになります なぜならそれが、活動銀河中心核でみられるエネルギー現象を 抑制しているからです
もしこのような目に見えないブラックホールを 銀河中心に探すとしたら 一番適切なのは我々の銀河「天の川」でです これは天の川銀河の中心部を 広角撮影した写真です 星の帯が見えています 私たちが、平たく、円盤のような形の銀河に 住んでいるからです 私たちはその中間地帯に住んでいて、そこから中心方向を見ると 銀河面を形成する面、あるいは その線が見えるわけです
自分たちの銀河を研究する利点は 単にそれが自分たちに一番近い 銀河中心だからです その次に近い銀河でも 100倍遠くにありますから 他のどこよりも自分たちの銀河のほうが 細部を見られるのです そしてこれからお見せしますが、どれくらい細部が見えるかが 研究の鍵なのです
さて、天文学者はどうやって小さな空間に大質量があると 証明するのでしょうか? それをこれからご覧に入れます その方法は、恒星がブラックホールの周りを回るのを 観察することです 恒星は、惑星が太陽の周りを回るのと同じように ブラックホールの周りを回ります 重力による引力で 物体の周回軌道が決まります もしそこに大質量がないとすれば、星は飛び去ってしまうか、 もっとゆっくり周回するでしょう なぜならどう周回するかを決めるのは 軌道内にある質量だからです
ちょうどいいですよね なぜなら私の仕事は 小さな空間に大質量があると証明することですから つまり星が周回する速度がわかれば、質量がわかります そして軌道の大きさがわかれば半径がわかる だから私は、銀河中心に できる限り近い恒星を見たいのです なるだけ小さい領域に、質量があることを示したいのですから つまり、なるべく詳細な姿を見たいということです だからこの研究のために世界最大の望遠鏡を 使っています
これはケック天文台です 10メートルの主鏡の 望遠鏡が二つあり、だいたいテニスコートくらいの 半径があります これは素晴らしいモノです なぜなら、巨大望遠鏡の公約は 「大きければ大きいほど 細部が見える」だからです しかし、こういう地上にある全ての望遠鏡は 公約を果たすには少しばかり問題があります それは大気のせいです 大気のおかげで私たちが地球に生存でき すばらしいものです しかし大気を通して天文光源をみる天文学者には かなりの問題をもたらします
ちょうど小川の水を通して 底にある小石を 見るようなものです 水底の小石を見ていると 水の流れがずっと乱れを起こし続けていて 流れの底にある小石を見るのはとても困難です 同じような理屈で、絶え間なく動いている 大気を通して天文光源を観測するのは 非常に困難なのです
そこで私はキャリアの多くの時間を 大気のゆらぎを補正し、明瞭な画像を得ることに費やしています 大体20倍ほど結果が向上します もし生活を20倍改善できる方法がわかれば ライフスタイルも 全然ちがうものになりますよね 給料にしても そうでしょう 子供のことにしても そうでしょう
お見せしている動画が、私たちが使っている 補償光学系とよばれる技術の例です ご覧の映像で その技術を使わなかった場合に見える映像、つまり 普通に見える星の映像が見えます 囲みの部分は銀河の中心にセットされていて そこにブラックホールがあるはずです 補正なしには星が見えません 補償光学技術を使うと、とたんに星が見え始めます この技術で、望遠鏡の光学システムに 大気のゆらぎに対抗して持続的に 変形する鏡を導入してあります 望遠鏡に、とっても素敵なメガネがついているわけね
ここから何枚かのスライドでは、あの小さな四角の部分に 注目します 他の部分も見えていますが 小さな四角の中にある星にだけ 注目して見てみます 私は恒星がどう動いたかを知りたいのですが この研究では、恒星が非常に大きく 動いています この研究を15年間やっていますが 恒星が周回運動しているのを観察してきました
大抵の天文学者にはお気に入りの恒星があり、 今日の私のお気に入りはこれです SO-2 世界で一番好きな恒星です たった15年で一周してくれたから それがどれくらい短いかというと 太陽だと銀河中心を一周するには2億年かかります これまで知られていた星で、銀河中心に一番近い星で 500年かかります この星は人の一生以内の時間で一周する これは有り難いことです
これがこの研究の鍵で、恒星の軌道から この非常に小さい半径に、どれだけの質量があるかがわかります 次の図では、この研究以前に 銀河中心の質量を閉じ込めることができると考えていた 大きさを示しています 以前から、この円の中に太陽の400万倍の 質量があることがわかっていました ご覧のように、円の中には様々なものが存在しました 恒星がたくさんあります つまり、様々なものを大量に詰め込むことができるので 銀河中心に超大質量ブラックホールがあるという説意外にも 多くの他の選択肢がありました
しかし我々の研究により 同じ質量が、これまでの1千万分の1という 遥かに小さい空間にあることがわかりました だからそこに超大質量 ブラックホールが存在すると証明できたのです どれくらい小さいかというと だいたい太陽系全体くらいの大きさです つまり太陽の400万倍の質量が、太陽系と 同じ大きさに詰まっているのです
つまり広告どおりよね でしょ? 私の仕事はそれをシュヴァルツシルト半径まで縮めることだと言いましたが 実際はまだそこまではいっていません しかしこの質量の集中を 説明する方法はこれ以外にないのです そして、これは私たちの銀河中心に超大質量 ブラックホールがあるという証拠であるだけでなく 全ての銀河にもあるという証拠でもあります では次はどうなるか? 実際のところ これが現在の技術でできる最大限だと思います そこで問題に取り組んでみましょう
つまり、今日ここで簡単にお話したのは 銀河中心に関して 現在 ご紹介できるもので もっともエキサイティングなことですが つまり銀河中心には 超大質量ブラックホールがある、あるいは そう信じているのです この研究で面白いのは もし銀河中心に超大質量ブラックホールが あった場合の結果に関する仮説を いくつか検討した結果 それらのどれ一つとして 実際に観察できるものと合致しないということです 面白いですよね
例を二つお示しします 質問:「銀河中心に 長期間存在する古い星は、ブラックホールとの 長期間の相互作用で、どうなっているだろうか?」 予想するのは、古い恒星が ブラックホールの周りに集まっている状態でしょう ブラックホールの近くに古い恒星がたくさんあるに違いない
同様に、あるいは対照的に、若い恒星は そこには存在するはずがない ブラックホールは、星の揺籃に関しては、親切な隣人ではありません 恒星ができるには、塊になるための非常に大きなガスと塵のボールが必要です それは非常に壊れやすい ブラックホールは何をすると思います? ガスを奪い去ってしまいます 一方の端を他方よりずっと強い力で引き寄せ 雲がちぎれてしまいます 実は、星の誕生はこういう場所では起きないだろうと予測していました
若い恒星はそこにあるはずがない 実際はどうだったか? 今日はお見せしなかった観測結果によって どの恒星が古く、どの恒星が若いかを知ることができます 赤いのが古い恒星で 青いのは若いやつです 黄色は、まだよくわかっていません もう、びっくりな状態がわかりますよね 古い恒星はわずかしかなく 若い恒星がたくさんあります 予想と全く逆だったのです
面白いですよね 現在、このことを私たちは解明しようとしています この結果のミステリー この矛盾をどう解決するのか 実際、私の院生が 今日この瞬間も、ハワイの あの望遠鏡で観測していて 次の段階の なぜ若い恒星が多く 古い恒星は少ないのかを調べる 段階に行こうとしています さらに進歩するには、もっとずっと遠い恒星の軌道を 調べる必要があります そのためには、おそらく現在よりもっともっと 高度な技術が必要です
なぜなら、実は、私は大気のゆらぎの補正を していますが、実際はエラーの 半分くらいしか補正できていないのです このために大気へ向けてレーザーを投射していますが さらにレーザーを増やせば 残りを補正できるでしょう これから数年間の内に、それができれば良いと思います さらいもっと長いタイムスパンでは さらに大きな望遠鏡を建設したいのです なぜなら天文学では「大きいことはいいこと」なのです
だから30メートルの望遠鏡を建設したい それを使えば、さらにもっと銀河中心に近い 恒星を観測することができるでしょう そしてアインシュタインの 一般相対性理論の一部を検証し 銀河の形成に関する宇宙論を検証したいのです この研究の未来は とても刺激的です
まとめますが、ご覧に入れる動画は これらの軌道が三次元空間で どう動いているかを示したものです そして、他のことはともかく ご理解いただきたいのは:(1)銀河中心に 超大質量ブラックホールが存在すること それは宇宙全体に存在し 私たちはそれに取り組んで、それらが我々の物理学世界に どう組み込めるかを説明しなくてはならないこと
(2)超大質量ブラックホールが どう相互作用するかを観測し あるいは、銀河の形成に果たす役割と その方法を理解するかもしれないこと
そして最後になりましたが これらの結果のどれ一つとして 技術の最前線で果たされた 莫大な進歩なしには起こり得なかったこと そしてその分野は非常に早く進歩していて もっと将来性があることです どうもありがとうございました (拍手)
品詞分類
- 主語
- 動詞
- 助動詞
- 準動詞
- 関係詞等
TED 日本語
TED Talks
関連動画
謎に包まれた土星の衛星タイタンを調べて生命の起源に関して何がわかるかエリザベス・ジビ・タートル
2020.09.17宇宙に関する理解に疑問を抱かせる珍しい銀河ブーチン・ムトゥル・パクディル
2018.09.18宇宙人はどこにいるのでしょう?スティーヴン・ウェッブ
2018.08.16他の恒星系からの初の訪問者オウムアムアカレン・J・ミーチ
2018.07.19地球上で最も火星によく似た場所アルマンド・アズア・ブストス
2017.10.05あなたは皆既日食を是非体験すべきであるデイヴィッド・バロン
2017.08.10ブラックホールの写真を撮影するケイティ・バウマン
2017.04.28地球を周回している宇宙ゴミを片付けましょうナタリー・パネク
2017.01.05地球外生命を宿しているかもしれない1つの惑星と3つの衛星ジェームズ・グリーン
2016.09.07惑星が生命を育むために必要なものデイヴ・ブレイン
2016.09.04火星に移住する子供達が生き抜く方法スティーブン・ペトラネック
2016.05.05宇宙でもっとも神秘的な星タベサ・ボヤジアン
2016.04.29重力波発見が意味することアラン・アダムス
2016.03.10他の惑星の生命を見つけ出す方法アオマワ・シールズ
2016.01.28物理学は終焉に達したのか?ハリー・クリフ
2016.01.26火星は予備の地球ではないルシアン・ウォーコウィッチ
2016.01.14
洋楽 おすすめ
RECOMMENDS
洋楽歌詞
ステイザ・キッド・ラロイ、ジャスティン・ビーバー
洋楽最新ヒット2021.08.20スピーチレス~心の声ナオミ・スコット
洋楽最新ヒット2019.05.23シェイプ・オブ・ユーエド・シーラン
洋楽人気動画2017.01.30フェイデッドアラン・ウォーカー
洋楽人気動画2015.12.03ウェイティング・フォー・ラヴアヴィーチー
洋楽人気動画2015.06.26シー・ユー・アゲインウィズ・カリファ
洋楽人気動画2015.04.06シュガーマルーン5
洋楽人気動画2015.01.14シェイク・イット・オフテイラー・スウィフト
ポップス2014.08.18オール・アバウト・ザット・ベースメーガン・トレイナー
ポップス2014.06.11ストーリー・オブ・マイ・ライフワン・ダイレクション
洋楽人気動画2013.11.03コール・ミー・メイビーカーリー・レイ・ジェプセン
洋楽人気動画2012.03.01美しき生命コールドプレイ
洋楽人気動画2008.08.04バッド・デイ~ついてない日の応援歌ダニエル・パウター
洋楽人気動画2008.05.14サウザンド・マイルズヴァネッサ・カールトン
洋楽人気動画2008.02.19イッツ・マイ・ライフボン・ジョヴィ
ロック2007.10.11アイ・ウォント・イット・ザット・ウェイバックストリート・ボーイズ
洋楽人気動画2007.09.14マイ・ハート・ウィル・ゴー・オンセリーヌ・ディオン
洋楽人気動画2007.07.12ヒーローマライア・キャリー
洋楽人気動画2007.03.21オールウェイズ・ラヴ・ユーホイットニー・ヒューストン
洋楽人気動画2007.02.19オネスティビリー・ジョエル
洋楽人気動画2005.09.16