
TED日本語
TED Talks(英語 日本語字幕付き動画)
TED日本語 - クレイグ・ベンター:「人工生命」について発表する
TED Talks
「人工生命」について発表する
Craig Venter unveils "synthetic life"
クレイグ・ベンター
内容
クレイグ・ベンターのチームが歴史的な発表を行いました。完全に機能する、人工のDNAによってコントロールされた自己増殖する細胞を初めて作り出したのです。どのようにしてこれを達成したのか、この成果が科学の新しい時代の幕開けとなる理由を語ります。
字幕
SCRIPT
Script
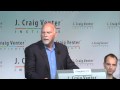
We're here today to announce the first synthetic cell, a cell made by starting with the digital code in the computer, building the chromosome from four bottles of chemicals, assembling that chromosome in yeast, transplanting it into a recipient bacterial cell and transforming that cell into a new bacterial species. So this is the first self-replicating species that we've had on the planet whose parent is a computer. It also is the first species to have its own website encoded in its genetic code. But we'll talk more about the watermarks in a minute.
This is a project that had its inception 15 years ago when our team then -- we called the institute TIGR -- was involved in sequencing the first two genomes in history. We did Haemophilus influenzae and then the smallest genome of a self-replicating organism, that of Mycoplasma genitalium. And as soon as we had these two sequences we thought, if this is supposed to be the smallest genome of a self-replicating species, could there be even a smaller genome? Could we understand the basis of cellular life at the genetic level? It's been a 15-year quest just to get to the starting point now to be able to answer those questions, because it's very difficult to eliminate multiple genes from a cell. You can only do them one at a time. We decided early on that we had to take a synthetic route, even though nobody had been there before, to see if we could synthesize a bacterial chromosome so we could actually vary the gene content to understand the essential genes for life. That started our 15-year quest to get here.
But before we did the first experiments, we actually asked Art Caplan's team at the University of Pennsylvania to undertake a review of what the risks, the challenges, the ethics around creating new species in the laboratory were because it hadn't been done before. They spent about two years reviewing that independently and published their results in Science in 1999. Ham and I took two years off as a side project to sequence the human genome, but as soon as that was done we got back to the task at hand.
In 2002, we started a new institute, the Institute for Biological Energy Alternatives, where we set out two goals: One, to understand the impact of our technology on the environment, and how to understand the environment better, and two, to start down this process of making synthetic life to understand basic life. In 2003, we published our first success. So Ham Smith and Clyde Hutchison developed some new methods for making error-free DNA at a small level. Our first task was a 5, 000-letter code bacteriophage, a virus that attacks only E. coli. So that was the phage phi X 174, which was chosen for historical reasons. It was the first DNA phage, DNA virus, DNA genome that was actually sequenced. So once we realized that we could make 5, 000-base pair viral-sized pieces, we thought, we at least have the means then to try and make serially lots of these pieces to be able to eventually assemble them together to make this mega base chromosome. So, substantially larger than we even thought we would go initially.
There were several steps to this. There were two sides: We had to solve the chemistry for making large DNA molecules, and we had to solve the biological side of how, if we had this new chemical entity, how would we boot it up, activate it in a recipient cell. We had two teams working in parallel: one team on the chemistry, and the other on trying to be able to transplant entire chromosomes to get new cells. When we started this out, we thought the synthesis would be the biggest problem, which is why we chose the smallest genome.
And some of you have noticed that we switched from the smallest genome to a much larger one. And we can walk through the reasons for that, but basically the small cell took on the order of one to two months to get results from, whereas the larger, faster-growing cell takes only two days. So there's only so many cycles we could go through in a year at six weeks per cycle. And you should know that basically 99, probably 99 percent plus of our experiments failed. So this was a debugging, problem-solving scenario from the beginning because there was no recipe of how to get there.
So,one of the most important publications we had was in 2007. Carole Lartigue led the effort to actually transplant a bacterial chromosome from one bacteria to another. I think philosophically, that was one of the most important papers that we've ever done because it showed how dynamic life was. And we knew, once that worked, that we actually had a chance if we could make the synthetic chromosomes to do the same with those. We didn't know that it was going to take us several years more to get there.
In 2008, we reported the complete synthesis of the Mycoplasma genitalium genome, a little over 500,000 letters of genetic code, but we have not yet succeeded in booting up that chromosome. We think in part, because of its slow growth and, in part, cells have all kinds of unique defense mechanisms to keep these events from happening. It turned out the cell that we were trying to transplant into had a nuclease, an enzyme that chews up DNA on its surface, and was happy to eat the synthetic DNA that we gave it and never got transplantations. But at the time, that was the largest molecule of a defined structure that had been made.
And so both sides were progressing, but part of the synthesis had to be accomplished or was able to be accomplished using yeast, putting the fragments in yeast and yeast would assemble these for us. It's an amazing step forward, but we had a problem because now we had the bacterial chromosomes growing in yeast. So in addition to doing the transplant, we had to find out how to get a bacterial chromosome out of the eukaryotic yeast into a form where we could transplant it into a recipient cell.
So our team developed new techniques for actually growing, cloning entire bacterial chromosomes in yeast. So we took the same mycoides genome that Carole had initially transplanted, and we grew that in yeast as an artificial chromosome. And we thought this would be a great test bed for learning how to get chromosomes out of yeast and transplant them. When we did these experiments, though, we could get the chromosome out of yeast but it wouldn't transplant and boot up a cell. That little issue took the team two years to solve.
It turns out, the DNA in the bacterial cell was actually methylated, and the methylation protects it from the restriction enzyme, from digesting the DNA. So what we found is if we took the chromosome out of yeast and methylated it, we could then transplant it. Further advances came when the team removed the restriction enzyme genes from the recipient capricolum cell. And once we had done that, now we can take naked DNA out of yeast and transplant it.
So last fall when we published the results of that work in Science, we all became overconfident and were sure we were only a few weeks away from being able to now boot up a chromosome out of yeast. Because of the problems with Mycoplasma genitalium and its slow growth about a year and a half ago, we decided to synthesize the much larger chromosome, the mycoides chromosome, knowing that we had the biology worked out on that for transplantation. And Dan led the team for the synthesis of this over one-million-base pair chromosome. But it turned out it wasn't going to be as simple in the end, and it set us back three months because we had one error out of over a million base pairs in that sequence.
So the team developed new debugging software, where we could test each synthetic fragment to see if it would grow in a background of wild type DNA. And we found that 10 out of the 11 100, 000-base pair pieces we synthesized were completely accurate and compatible with a life-forming sequence. We narrowed it down to one fragment; we sequenced it and found just one base pair had been deleted in an essential gene. So accuracy is essential. There's parts of the genome where it can not tolerate even a single error, and then there's parts of the genome where we can put in large blocks of DNA, as we did with the watermarks, and it can tolerate all kinds of errors. So it took about three months to find that error and repair it. And then early one morning, at 6 a.m. we got a text from Dan saying that, now, the first blue colonies existed.
So, it's been a long route to get here: 15 years from the beginning. We felt one of the tenets of this field was to make absolutely certain we could distinguish synthetic DNA from natural DNA. Early on, when you're working in a new area of science, you have to think about all the pitfalls and things that could lead you to believe that you had done something when you hadn't, and, even worse, leading others to believe it. So, we thought the worst problem would be a single molecule contamination of the native chromosome, leading us to believe that we actually had created a synthetic cell, when it would have been just a contaminant.
So early on, we developed the notion of putting in watermarks in the DNA to absolutely make clear that the DNA was synthetic. And the first chromosome we built in 2008 -- the 500, 000-base pair one -- we simply assigned the names of the authors of the chromosome into the genetic code, but it was using just amino acid single letter translations, which leaves out certain letters of the alphabet. So the team actually developed a new code within the code within the code. So it's a new code for interpreting and writing messages in DNA. Now, mathematicians have been hiding and writing messages in the genetic code for a long time, but it's clear they were mathematicians and not biologists because, if you write long messages with the code that the mathematicians developed, it would more than likely lead to new proteins being synthesized with unknown functions.
So the code that Mike Montague and the team developed actually puts frequent stop codons, so it's a different alphabet but allows us to use the entire English alphabet with punctuation and numbers. So, there are four major watermarks all over 1,000 base pairs of genetic code. The first one actually contains within it this code for interpreting the rest of the genetic code. So in the remaining information, in the watermarks, contain the names of, I think it's 46 different authors and key contributors to getting the project to this stage. And we also built in a website address so that if somebody decodes the code within the code within the code, they can send an email to that address. So it's clearly distinguishable from any other species, having 46 names in it, its own web address. And we added three quotations, because with the first genome we were criticized for not trying to say something more profound than just signing the work.
So we won't give the rest of the code, but we will give the three quotations. The first is, "To live, to err, to fall, to triumph and to recreate life out of life." It's a James Joyce quote. The second quotation is, "See things not as they are, but as they might be." It's a quote from the "American Prometheus" book on Robert Oppenheimer. And the last one is a Richard Feynman quote: "What I can not build, I can not understand." So, because this is as much a philosophical advance as a technical advance in science, we tried to deal with both the philosophical and the technical side.
The last thing I want to say before turning it over to questions is that the extensive work that we've done -- asking for ethical review, pushing the envelope on that side as well as the technical side -- this has been broadly discussed in the scientific community, in the policy community and at the highest levels of the federal government. Even with this announcement, as we did in 2003 -- that work was funded by the Department of Energy, so the work was reviewed at the level of the White House, trying to decide whether to classify the work or publish it. And they came down on the side of open publication, which is the right approach -- we've briefed the White House, we've briefed members of Congress, we've tried to take and push the policy issues in parallel with the scientific advances.
So with that, I would like to open it first to the floor for questions. Yes, in the back.
Reporter: Could you explain, in layman's terms, how significant a breakthrough this is please?
Craig Venter: Can we explain how significant this is? I'm not sure we're the ones that should be explaining how significant it is. It's significant to us. Perhaps it's a giant philosophical change in how we view life. We actually view it as a baby step in terms of, it's taken us 15 years to be able to do the experiment we wanted to do 15 years ago on understanding life at its basic level. But we actually believe this is going to be a very powerful set of tools and we're already starting in numerous avenues to use this tool.
We have, at the Institute, ongoing funding now from NIH in a program with Novartis to try and use these new synthetic DNA tools to perhaps make the flu vaccine that you might get next year. Because instead of taking weeks to months to make these, Dan's team can now make these in less than 24 hours. So when you see how long it took to get an H1N1 vaccine out, we think we can shorten that process quite substantially. In the vaccine area, Synthetic Genomics and the Institute are forming a new vaccine company because we think these tools can affect vaccines to diseases that haven't been possible to date, things where the viruses rapidly evolve, such with rhinovirus. Wouldn't it be nice to have something that actually blocked common colds? Or, more importantly, HIV, where the virus evolves so quickly the vaccines that are made today can't keep up with those evolutionary changes.
Also, at Synthetic Genomics, we've been working on major environmental issues. I think this latest oil spill in the Gulf is a reminder. We can't see CO2 -- we depend on scientific measurements for it and we see the beginning results of having too much of it -- but we can see pre-CO2 now floating on the waters and contaminating the beaches in the Gulf. We need some alternatives for oil. We have a program with Exxon Mobile to try and develop new strains of algae that can efficiently capture carbon dioxide from the atmosphere or from concentrated sources, make new hydrocarbons that can go into their refineries to make normal gasoline and diesel fuel out of CO2.
Those are just a couple of the approaches and directions that we're taking.
(Applause)
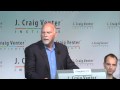
本日 発表致しますのは 初の人工細胞です 細胞は コンピュータのデジタルコードとして誕生し 4本の化学物質のボトルから 染色体が作られ その染色体はイースト菌内で組み立てられ レシピエントとなる細菌の細胞に 移植され その細胞が 別の種の細菌へと変化したのです つまり これはこの惑星上で初めての コンピュータを親にもつ 自己複製できる種なのです また 自分のWebサイトに エンコードした遺伝情報を公開した 初めての種でもあります それでは少し ウオーターマーク(目印)についてお話ししましょう
このプロジェクトの発端は 15年前に遡ります 我々のチームは その頃はTIGRと呼んでいましたが 歴史上初めて 二つのゲノムの 配列を決める仕事に取り組んでいました インフルエンザ菌 そして自己複製する生命体として最小である マイコプラズマ=ジェニタリウムです これらのゲノム配列を 決定し終えた後で考えたことは これが自己複製する種で最小の ゲノムであるとすれば さらに小さなゲノムは存在するのだろうか そして ゲノムのレベルで細胞の活動に関する基礎を 理解できないかということです 15年間の探究を経て この問いに答えるための スタートに やっとたどり着くことができました 細胞から複数の遺伝子を 除去することは非常に困難だからです 一度に一つずつ除去するしかないのです そこで早い段階で 人工的な手段を採ることにしました 誰も試していない方法ではありましたが 細菌の染色体を 合成できるのか確かめ どの遺伝子が必須であるかを理解するため 遺伝子の内容を様々に変えてみました それが15年の探究の 始まりだったのです
最初の実験を始める前に 我々は 当時ペンシルバニア大学にあったアート=カプランのチームに 検討を依頼しました 研究室で新しい種を作ることに対して どのようなリスクや課題 倫理問題が 存在するかという検討です 初めての試みだったからです アート=カプランは2年を費やして 独自に検討を行い 1999年にサイエンス誌に結果を発表しました ハムと私は2年間 この研究から離れ ヒトゲノムを解析する別のプロジェクトに参加していました しかし 結果が発表されるや否や 直ちに この仕事に戻りました
2002年に 新たな機関を設立しました それが生物代替エネルギー研究所です 目標は二つありました 一つ目は 我々の技術が 環境に与える影響を把握し 環境をより深く理解する手法を見つけること 二つ目は 人工生命を作る過程を経て 生命の基礎についての理解を 深めることです 2003年に 最初の成果を公開しました ハム=スミスとクライド=ハッチンソンが 小さなレベルで エラーのないDNAを作る 新しい手法を開発したのです 最初の仕事の対象は バクテリオファージの5000文字のコードでした これは大腸菌のみを攻撃するウイルスです ファージ ファイ X 174 を使用しましたが その選択には歴史的経緯があります 事実上 初めて解読された DNAファージであり DNAウイルス DNAゲノムだったのです 5000の塩基対でできている ウイルスサイズのピースを作成することが 可能であると判明したので これで道は開けたと考え このピースを大量に連続して作成することを試みました 最終目標は ピースを纏めて作成し このメガ塩基対の染色体を作成することでした これは当初想定していたより かなり大きなサイズでした
幾つかの段階がありました これには二つの課題がありました 大きなサイズのDNA分子を作るには 化学的な問題を解決すると共に 生物学的な問題も解決する必要がありました つまり この新たな化学物質を作り出せたとして それをレシピエントの細胞内で どのように起動し 活性化するかということです そこで 二つのチームが平行して作業を行いました 一つは化学チーム もう一つのチームは 染色体全体を 新しい細胞に移植するための 研究をしていました 研究を始めた当初は 合成が最大の課題になると考え 最小のゲノムを選択することにしました
お気づきのように 我々は最小のゲノムではなく より大きなゲノムを対象に変えました その理由をいくつかご紹介することは出来ますが 基本的に 小さな細胞では 結果が判明するまでに 1~2ヶ月の単位で時間がかかる一方 大きな細胞の場合は 成長が早く 二日ほどで結果が得られるからです その結果 1サイクル6週間で1年間に 何サイクルも結果を検討することができたのです 我々の行った実験の99パーセント 恐らく99パーセント以上が 失敗したことをご理解いただけるでしょう これは所謂デバッグ作業です つまり当初から 問題解決型のシナリオであったのです なぜなら 成功への道筋は 存在しなかったからです
最も重大は発表は2007年に 行ったものです キャラル=ラルティグのチームが 染色体を ある細菌から 別の細菌に移植することに成功したのです 振り返って考えると これは我々が発表した成果の中で 最も重要なものだったと思います 生命がいかにダイナミックなものかを示していたからです そして これが上手くゆけば 本当にチャンスがあるのだと考えていました 染色体を合成できれば あとは同じことをすれば良かったのです あと数年で達成できるのか それとも さらに年月を要するのかは分かっていませんでした
2008年に マイコプラズマ=ジェニタリウムのゲノム 50万文字を上回るゲノムコードを 完全に合成できたと発表しました しかし 染色体を起動することには成功していませんでした 理由として 一点目は成長の遅さ もう一点は 細胞がこのような事態を防ぐための様々な防衛システムを 持っているためだと考えました 我々が移植対象としていた細胞には 表面に ヌクレアーゼというDNA分解酵素があることが分かったのです 我々が与えた合成DNAを 好んで消化してしまっており 移植の障害となっていたのです しかし当時は それが過去に作成された 定義済みの構造体のうち 最大の分子でした
どちらの面でも進歩が見られました しかし一部の合成はイースト菌を使用し この物質をイースト菌に入れることで 我々に変わって合成を行ってくれるはずであり また それは達成せねばなりませんでした 前に進めることは喜びでしたが 問題もありました 細菌の染色体をイースト菌の中で育てていましたので 移植をおこなうためには 細菌の染色体を真核性のイースト菌から レシピエントに移植可能な形で取り出すには どうすれば良いのか明確にする 必要があったのです
そこで我々のチームは 完全な細菌の染色体を イースト菌の中で成長させ クローンを作成する 新技術を開発しました そこでキャロルが最初に移植に成功したのと同じ ミコイデスのゲノムを 人工染色体として イースト菌の中で成長させることを試みました イースト菌から染色体を取り出し 移植する方法を学ぶ上で 有効な試験台となると考えたのです そして実験を行いましたが イースト菌から染色体を取り出すことはできても それを移植し 細胞の中で起動させることはできませんでした この小さな問題の解決に2年費やしました
細菌の細胞に存在するDNAは メチル基と結合しており メチル化することによって 制限酵素による DNAの分解から守られていることが分かりました つまり イースト菌から染色体を取り出し メチル化することができれば 移植が可能になるということが分かったのです レシピエントであるマイコプラズマの細胞から 制限酵素の遺伝子を取り除くことに成功し 研究はさらに進歩しました いったん これに成功して以降 DNAをそのまま移植することが可能となりました
昨秋 我々は 成果をサイエンス誌に公表しました 我々は自信過剰となり イースト菌から取り出した染色体を あと数週間で 細胞内で起動させることが 実現可能であると考えていました 1年半前のマイコプラズマ=ジェニタリウムと その成長の遅さに関する問題が 1年半前に解決していたため さらに大きな染色体である ミコイデの染色体を 合成することにしました 移植に当たって 我々は生物学的にうまくいくと 考えていました ダンのチームが この百万の塩基対からなる染色体の 合成に挑みました しかしそれは後に それほど単純ではないことが分かったのです そして3ヶ月分 後戻りすることになりました 合成した配列において 百万の塩基対のうち 1塩基分間違いがあることが分かったのです
そこでダンのチームは新たなデバッグ用ソフトウエアを開発し 合成断片が野生型DNAの環境において 増えることができるかどうか テストを実施することにしました 合成した10万の塩基対のうち 割合にして 11セット中10セットは 生命を形成する配列と 完全に正確で 一致していました 一つの断片に焦点を絞り その配列を調べたところ 必須遺伝子の たった一つの塩基対が 欠落していることが分かりました このように 正確さが不可欠なのです ゲノムには たった一つのエラーも許されない部分がある一方 我々が目印として 長い配列のDNAを組み込んだように どんなエラーがあったとしても 問題がない部分が存在するのです このため エラーを発見して修正するのに 三ヶ月かかりました そしてある朝の6時にダンから 初めて青色のコロニーが見つかったと メッセージを受け取ったのです
大変長い道のりでした 15年を費やしました この分野の研究において 守るべき基本原則の一つは 人工合成したDNAと天然のDNAを 明確に見分けることができるようにすることだと 考えていました 科学の新しい分野に取り組む時 早い段階では あらゆる落とし穴について考慮する必要があります あるいは 達成していないことを 達成できたと思わせるようなものについて注意を払うべきです 最悪の場合 他人にもそれを信じさせてしまうことになります 最も考慮すべき問題は 自然に存在する染色体の たった一つの分子の混入によって 単なる混入に過ぎないというのに 細胞を合成することができたと 誤信してしまうことだと考えていました
そこで早い段階から DNAに目印を付けて そのDNAが合成のものであることが 明確になるようにしました 2008年に最初の染色体を 作った時は 50万塩基対の染色体に 染色体作成者の名前を 遺伝子コードとして 組み込みました しかし それはアミノ酸配列の 一文字表記を利用したものであり アルファベットの特定の文字が除外されたものでした このようにして 遺伝子コードの中に 別の遺伝子コードを埋め込みました DNAに新しいコードを 変換して書き込んだことになります 長い間 遺伝子コードにメッセージを書き込む仕事は 数学者が行ってきました 数学者は生物学者ではありません 数学者が作成したコードを使って 長いメッセージを書いたとすると 未知の機能を持った 新しいタンパク質の 合成につながることでしょう
そこで マイク=モンタギューのチームが開発したコードでは 度々 終始コドンを加えています 異なった体系のアルファベッドですが これによって すべての英文字と 句読点 数字を使用できるようになりました 膨大な遺伝子コードの中で 主に使用している目印は4つあります 一つ目は遺伝子コードの残りの部分を 解読するためのコードを 含んだものです 目印の中には その他の情報として 作者や このプロジェクトを 成功に導いた主立った貢献者の 名前が含まれています 確か46人だったと思います 名前だけではなく Webサイトのアドレスも記してあります もしコードに含まれるコードを利用して 誰かがコードを解読したとしたら このアドレスにメールを送ることができます つまり 他の種とは明確に 区別できるということです 46名の名前を持ち ウエブのアドレスが記されているのですから 引用句も3つ記してあります なぜなら 最初のゲノムの時には 意味深げなことを言わずに 作品にサインをしただけだと 批判されたからです
そこで 残りのコードの場所はお伝えしませんが 代わりに3つの引用句をご紹介します 一つ目は "To live, to err, to fall, to triumph, and to recreate life out of life.'' ジェームス=ジョイスの言葉です 二つ目は ''See things, not as they are, but as they might be.'' ロバート=オッペンハイマーの著書 「アメリカのプロメテウス」からの引用です 最後はリチャード=ファイマンの言葉で 'What I cannot build, I cannot understand.'' 今回の成果は科学における技術的進歩であるとともに 哲学的な進歩でもあるという観点から 技術的側面に加えて 哲学的側面にも 対応しようと試みたというわけです
質疑に入る前に 一言付け加えたいと思います 我々は多岐にわたる活動を 行ってきました これに関して倫理的な検討を求め 技術的な面と同様に その面においても限界を広げようと試みました 科学界および政界においても 広く議論がなされました 連邦政府でも高レベルでの検討がなされています 今回の発表についても 2003年の発表と同様でした エネルギー省から資金を得ていましたので ホワイトハウスのレベルにおいて 極秘にしておくか 公開するかの決定について ホワイトハウスのレベルでの検討が行われたのです そして公開という正しい形で 実を結んだのです ホワイトハウスを説得し 何人もの議員も説得しました 科学的な進歩を続けるとともに 政治的な問題についても 解決しようと努めてきたのです
それでは ここで 皆様からの質問を受けたいと思います では 後ろの方
記者:今回の発表がどのくらい革命的であるか 素人にも分かるように説明していただけるでしょうか
クレイグ:重要性についての質問ですね 重要性について私が説明すべき立場にあるかどうかは分かりません これは全員にとって重要なことです 我々の生命に対するとらえ方が 哲学的に大きく変わるでしょう 基本的なレベルで生命を理解すべく 15年前に研究を開始しました 15年間かけて 到達した現在の段階は まだ ほんの手始めでしかないと思います しかし この成果は今後 非常に有効なツールとなると確信しています すでに このツールを使った 研究をいくつか 開始しています
我々の研究機関において 現在 国立衛生研究所から資金提供を受け ノバルティス社と共同で これらの合成DNAのツールを使用した プロジェクトを行っています おそらく来年には インフルエンザの ワクチンを提供できると思います 以前であれば数週間から数ヶ月を要していたことを ダンのチームは24時間以内で完了できる ようになったのです H1N1ワクチンができるまでどのくらい必要かと考えると 大幅に必要な時間を短縮できると 考えられるのです ワクチンの分野では シンセティック=ゲノミクス社と共同で 会社を設立しようとしてるところです その理由は このツールによって 今までは対応ができないと思われていた病気のワクチン 進化が非常に早いライノウイルスなどのワクチンを 開発できると考えているためです 普通の風邪を防ぐ方法が見つかったら素晴らしいでしょう HIVだと もっと素晴らしいはずです こういった病気では ウイルスが急速に進化するために 現在の方法ではワクチンが ウイルスの進化による変化に 追いついていないのです
また シンセティック=ゲノミクス社では 重要な環境問題についても 取り組んでいます メキシコ湾での石油流出は 我々への警告です CO2は目に見えず 計測には科学的手法が必要です そして CO2の排出量が増えすぎている 状況を目の当たりにしています しかし現在 CO2の前段階である 石油が海上や 湾岸地域の海岸を汚染しているのです 石油の代替となるものが 必要です エクソン=モービル社と共同で 大気中や 濃縮された物質から 効果的に二酸化炭素を吸収する 新種の藻を開発しようとしています エクソンの精油所で その藻が発生した炭化水素から CO2を含まないガソリンや ディーゼル燃料が生成されるのです
これらは我々が現在行っている 研究アプローチの例に過ぎません
(拍手)
品詞分類
- 主語
- 動詞
- 助動詞
- 準動詞
- 関係詞等
TED 日本語
TED Talks
関連動画
プラスチックを食べる細菌モーガン・ヴェイグ
2019.06.24微生物の世界へようこそ ― 自宅にも、そしてあなたの顔にもアン・マデン
2017.08.24CRISPRについて、みんなが知るべきことエレン・ヨルゲンセン
2016.10.24自然に潜む驚異的な力を生かす方法オーデッド・ショゼヨフ
2016.10.18自分でDNA検査ができる時代の到来セバスチャン・クレイベス
2016.10.13リンゴから耳を作るマッドサイエンティストアンドリュー・ペリング
2016.07.081つの生物種全体を永久に変えてしまう遺伝子編集技術ジェニファー・カーン
2016.06.02ゲノムを読んで人間を作る方法リッカルド・サバティーニ
2016.05.24宇宙での生存に備えて人類が進化する方法リサ・ニップ
2016.04.21干ばつに耐えられる農作物の作り方ジル・ファラント
2016.02.09DNA編集が可能な時代、使い方は慎重にジェニファー・ダウドナ
2015.11.12テクノロジーとバイオロジーを融合したデザインネリ・オックスマン
2015.10.29アニメーションで科学者の仮説を試す方法ジャネット・イワサ
2014.08.07二人の若き科学者、バクテリアでプラスチックを分解
2013.07.18毛長マンモスを復活させよう!ヘンドリック・ポイナー
2013.05.30私たちを取り巻く細菌と住環境のデザインジェシカ・グリーン
2013.03.25
洋楽 おすすめ
RECOMMENDS
洋楽歌詞
ダイナマイトビーティーエス
洋楽最新ヒット2020.08.20ディス・イズ・ミーグレイテスト・ショーマン・キャスト
洋楽人気動画2018.01.11グッド・ライフGイージー、ケラーニ
洋楽人気動画2017.01.27ホワット・ドゥ・ユー・ミーン?ジャスティン・ビーバー
洋楽人気動画2015.08.28ファイト・ソングレイチェル・プラッテン
洋楽人気動画2015.05.19ラヴ・ミー・ライク・ユー・ドゥエリー・ゴールディング
洋楽人気動画2015.01.22アップタウン・ファンクブルーノ・マーズ、マーク・ロンソン
洋楽人気動画2014.11.20ブレイク・フリーアリアナ・グランデ
洋楽人気動画2014.08.12ハッピーファレル・ウィリアムス
ポップス2014.01.08カウンティング・スターズワンリパブリック
ロック2013.05.31ア・サウザンド・イヤーズクリスティーナ・ペリー
洋楽人気動画2011.10.26ユー・レイズ・ミー・アップケルティック・ウーマン
洋楽人気動画2008.05.30ルーズ・ユアセルフエミネム
洋楽人気動画2008.02.21ドント・ノー・ホワイノラ・ジョーンズ
洋楽人気動画2008.02.15オンリー・タイムエンヤ
洋楽人気動画2007.10.03ミス・ア・シングエアロスミス
ロック2007.08.18タイム・トゥ・セイ・グッバイサラ・ブライトマン
洋楽人気動画2007.06.08シェイプ・オブ・マイ・ハートスティング
洋楽人気動画2007.03.18ウィ・アー・ザ・ワールド(U.S.A. フォー・アフリカ)マイケル・ジャクソン
洋楽人気動画2006.05.14ホテル・カリフォルニアイーグルス
ロック2005.07.06