
TED日本語
TED Talks(英語 日本語字幕付き動画)
TED日本語 - マーチン・ハンジク: 生命と非生命の境界線
TED Talks
生命と非生命の境界線
The line between life and not-life
マーチン・ハンジク
Martin Hanczyc
内容
マーチン・ハンジクの研究室ではプロトセルという化学物質の塊を作り、生命のような挙動を観察しています。この研究は、生命が地球上で誕生したときの姿に関わり、また他の星で誕生するときの姿も示唆するものです。
字幕
SCRIPT
Script
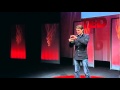
So historically there has been a huge divide between what people consider to be non-living systems on one side, and living systems on the other side. So we go from, say, this beautiful and complex crystal as non-life, and this rather beautiful and complex cat on the other side. Over the last hundred and fifty years or so, science has kind of blurred this distinction between non-living and living systems, and now we consider that there may be a kind of continuum that exists between the two. We'll just take one example here: a virus is a natural system, right? But it's very simple. It's very simplistic. It doesn't really satisfy all the requirements, it doesn't have all the characteristics of living systems and is in fact a parasite on other living systems in order to, say, reproduce and evolve.
But what we're going to be talking about here tonight are experiments done on this sort of non-living end of this spectrum -- so actually doing chemical experiments in the laboratory, mixing together nonliving ingredients to make new structures, and that these new structures might have some of the characteristics of living systems. Really what I'm talking about here is trying to create a kind of artificial life.
So what are these characteristics that I'm talking about? These are them. We consider first that life has a body. Now this is necessary to distinguish the self from the environment. Life also has a metabolism. Now this is a process by which life can convert resources from the environment into building blocks so it can maintain and build itself. Life also has a kind of inheritable information. Now we, as humans, we store our information as DNA in our genomes and we pass this information on to our offspring. If we couple the first two -- the body and the metabolism -- we can come up with a system that could perhaps move and replicate, and if we coupled these now to inheritable information, we can come up with a system that would be more lifelike, and would perhaps evolve. And so these are the things we will try to do in the lab, make some experiments that have one or more of these characteristics of life.
So how do we do this? Well, we use a model system that we term a protocell. You might think of this as kind of like a primitive cell. It is a simple chemical model of a living cell, and if you consider for example a cell in your body may have on the order of millions of different types of molecules that need to come together, play together in a complex network to produce something that we call alive. In the laboratory what we want to do is much the same, but with on the order of tens of different types of molecules -- so a drastic reduction in complexity, but still trying to produce something that looks lifelike. And so what we do is, we start simple and we work our way up to living systems. Consider for a moment this quote by Leduc, a hundred years ago, considering a kind of synthetic biology: "The synthesis of life, should it ever occur, will not be the sensational discovery which we usually associate with the idea." That's his first statement. So if we actually create life in the laboratories, it's probably not going to impact our lives at all.
"If we accept the theory of evolution, then the first dawn of synthesis of life must consist in the production of forms intermediate between the inorganic and the organic world, or between the non-living and living world, forms which possess only some of the rudimentary attributes of life" -- so, the ones I just discussed -- "to which other attributes will be slowly added in the course of development by the evolutionary actions of the environment." So we start simple, we make some structures that may have some of these characteristics of life, and then we try to develop that to become more lifelike. This is how we can start to make a protocell. We use this idea called self-assembly. What that means is, I can mix some chemicals together in a test tube in my lab, and these chemicals will start to self-associate to form larger and larger structures. So say on the order of tens of thousands, hundreds of thousands of molecules will come together to form a large structure that didn't exist before. And in this particular example, what I took is some membrane molecules, mixed those together in the right environment, and within seconds it forms these rather complex and beautiful structures here. These membranes are also quite similar, morphologically and functionally, to the membranes in your body, and we can use these, as they say, to form the body of our protocell.
Likewise, we can work with oil and water systems. As you know, when you put oil and water together, they don't mix, but through self-assembly we can get a nice oil droplet to form, and we can actually use this as a body for our artificial organism or for our protocell, as you will see later. So that's just forming some body stuff, right? Some architectures. What about the other aspects of living systems? So we came up with this protocell model here that I'm showing. We started with a natural occurring clay called montmorillonite. This is natural from the environment, this clay. It forms a surface that is, say, chemically active. It could run a metabolism on it. Certain kind of molecules like to associate with the clay. For example, in this case, RNA, shown in red -- this is a relative of DNA, it's an informational molecule -- it can come along and it starts to associate with the surface of this clay. This structure, then, can organize the formation of a membrane boundary around itself, so it can make a body of liquid molecules around itself, and that's shown in green here on this micrograph. So just through self-assembly, mixing things together in the lab, we can come up with, say, a metabolic surface with some informational molecules attached inside of this membrane body, right?
So we're on a road towards living systems. But if you saw this protocell, you would not confuse this with something that was actually alive. It's actually quite lifeless. Once it forms, it doesn't really do anything. So, something is missing. Some things are missing. So some things that are missing is, for example, if you had a flow of energy through a system, what we'd want is a protocell that can harvest some of that energy in order to maintain itself, much like living systems do. So we came up with a different protocell model, and this is actually simpler than the previous one. In this protocell model, it's just an oil droplet, but a chemical metabolism inside that allows this protocell to use energy to do something, to actually become dynamic, as we'll see here. You add the droplet to the system. It's a pool of water, and the protocell starts moving itself around in the system. Okay? Oil droplet forms through self-assembly, has a chemical metabolism inside so it can use energy, and it uses that energy to move itself around in its environment.
As we heard earlier, movement is very important in these kinds of living systems. It is moving around, exploring its environment, and remodeling its environment, as you see, by these chemical waves that are forming by the protocell. So it's acting, in a sense, like a living system trying to preserve itself. We take this same moving protocell here, and we put it in another experiment, get it moving. Then I'm going to add some food to the system, and you'll see that in blue here, right? So I add some food source to the system. The protocell moves. It encounters the food. It reconfigures itself and actually then is able to climb to the highest concentration of food in that system and stop there. Alright? So not only do we have this system that has a body, it has a metabolism, it can use energy, it moves around. It can sense its local environment and actually find resources in the environment to sustain itself.
Now, this doesn't have a brain, it doesn't have a neural system. This is just a sack of chemicals that is able to have this interesting and complex lifelike behavior. If we count the number of chemicals in that system, actually, including the water that's in the dish, we have five chemicals that can do this. So then we put these protocells together in a single experiment to see what they would do, and depending on the conditions, we have some protocells on the left that are moving around and it likes to touch the other structures in its environment. On the other hand we have two moving protocells that like to circle each other, and they form a kind of a dance, a complex dance with each other. Right? So not only do individual protocells have behavior, what we've interpreted as behavior in this system, but we also have basically population-level behavior similar to what organisms have. So now that you're all experts on protocells, we're going to play a game with these protocells. We're going to make two different kinds. Protocell A has a certain kind of chemistry inside that, when activated, the protocell starts to vibrate around, just dancing. So remember, these are primitive things, so dancing protocells, that's very interesting to us. (Laughter)
The second protocell has a different chemistry inside, and when activated, the protocells all come together and they fuse into one big one. Right? And we just put these two together in the same system. So there's population A, there's population B, and then we activate the system, and protocell Bs, they're the blue ones, they all come together. They fuse together to form one big blob, and the other protocell just dances around. And this just happens until all of the energy in the system is basically used up, and then, game over. So then I repeated this experiment a bunch of times, and one time something very interesting happened. So, I added these protocells together to the system, and protocell A and protocell B fused together to form a hybrid protocell AB. That didn't happen before. There it goes. There's a protocell AB now in this system. Protocell AB likes to dance around for a bit, while protocell B does the fusing, okay?
But then something even more interesting happens. Watch when these two large protocells, the hybrid ones, fuse together. Now we have a dancing protocell and a self-replication event. Right. (Laughter) Just with blobs of chemicals, again. So the way this works is, you have a simple system of five chemicals here, a simple system here. When they hybridize, you then form something that's different than before, it's more complex than before, and you get the emergence of another kind of lifelike behavior which in this case is replication.
So since we can make some interesting protocells that we like, interesting colors and interesting behaviors, and they're very easy to make, and they have interesting lifelike properties, perhaps these protocells have something to tell us about the origin of life on the Earth. Perhaps these represent an easily accessible step,one of the first steps by which life got started on the early Earth. Certainly, there were molecules present on the early Earth, but they wouldn't have been these pure compounds that we worked with in the lab and I showed in these experiments. Rather, they'd be a real complex mixture of all kinds of stuff, because uncontrolled chemical reactions produce a diverse mixture of organic compounds. Think of it like a primordial ooze, okay? And it's a pool that's too difficult to fully characterize, even by modern methods, and the product looks brown, like this tar here on the left. A pure compound is shown on the right, for contrast.
So this is similar to what happens when you take pure sugar crystals in your kitchen, you put them in a pan, and you apply energy. You turn up the heat, you start making or breaking chemical bonds in the sugar, forming a brownish caramel, right? If you let that go unregulated, you'll continue to make and break chemical bonds, forming an even more diverse mixture of molecules that then forms this kind of black tarry stuff in your pan, right, that's difficult to wash out. So that's what the origin of life would have looked like. You needed to get life out of this junk that is present on the early Earth,four,4.5 billion years ago. So the challenge then is, throw away all your pure chemicals in the lab, and try to make some protocells with lifelike properties from this kind of primordial ooze.
So we're able to then see the self-assembly of these oil droplet bodies again that we've seen previously, and the black spots inside of there represent this kind of black tar -- this diverse, very complex, organic black tar. And we put them into one of these experiments, as you've seen earlier, and then we watch lively movement that comes out. They look really good, very nice movement, and also they appear to have some kind of behavior where they kind of circle around each other and follow each other, similar to what we've seen before -- but again, working with just primordial conditions, no pure chemicals. These are also, these tar-fueled protocells, are also able to locate resources in their environment. I'm going to add some resource from the left, here, that defuses into the system, and you can see, they really like that. They become very energetic, and able to find the resource in the environment, similar to what we saw before. But again, these are done in these primordial conditions, really messy conditions, not sort of sterile laboratory conditions. These are very dirty little protocells, as a matter of fact. (Laughter) But they have lifelike properties, is the point.
So, doing these artificial life experiments helps us define a potential path between non-living and living systems. And not only that, but it helps us broaden our view of what life is and what possible life there could be out there -- life that could be very different from life that we find here on Earth. And that leads me to the next term, which is "weird life." This is a term by Steve Benner. This is used in reference to a report in 2007 by the National Research Council in the United States, wherein they tried to understand how we can look for life elsewhere in the universe, okay, especially if that life is very different from life on Earth. If we went to another planet and we thought there might be life there, how could we even recognize it as life?
Well, they came up with three very general criteria. First is -- and they're listed here. The first is, the system has to be in non-equilibrium. That means the system can not be dead, in a matter of fact. Basically what that means is, you have an input of energy into the system that life can use and exploit to maintain itself. This is similar to having the Sun shining on the Earth, driving photosynthesis, driving the ecosystem. Without the Sun, there's likely to be no life on this planet. Secondly, life needs to be in liquid form, so that means even if we had some interesting structures, interesting molecules together but they were frozen solid, then this is not a good place for life. And thirdly, we need to be able to make and break chemical bonds. And again this is important because life transforms resources from the environment into building blocks so it can maintain itself.
Now today, I told you about very strange and weird protocells -- some that contain clay, some that have primordial ooze in them, some that have basically oil instead of water inside of them. Most of these don't contain DNA, but yet they have lifelike properties. But these protocells satisfy these general requirements of living systems. So by making these chemical, artificial life experiments, we hope not only to understand something fundamental about the origin of life and the existence of life on this planet, but also what possible life there could be out there in the universe. Thank you. (Applause)
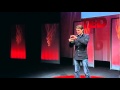
歴史的に見ると 生命ではないと考えられるものと 生命と考えられるものとは対極に置かれ 大きく隔たっていました この美しくて複雑な 結晶は非生命の側にあり 美しくて複雑な猫が反対側になります これまで150年ほどの科学によって 非生命と生命との区別は はっきりしなくなり いまや両者の間は切れ目なく 続いているかのようです 一例をあげましょう ウィルスは自然に存在しますね ただし単純きわまりないものです 生命としての 必要条件すら満たしません 生命の特徴を全ては満たさず 他の生命に寄生することで 繁殖したり進化を遂げます
今夜のお話では この並びの中では非生命寄りに 位置づけられる実験を紹介します 実験室で行う化学の実験です 非生命体の材料を混合して 新しい構造を作り出し その構造が 生体系の特徴の一部を有するのです これはつまり ある種の人工生命を作る試みです
どんな特徴に注目しているかというと こんな特徴です まず 生命には肉体があります 肉体は自己を 環境と区別するために必要です また生命は代謝します これは環境から取り込んだ資源を 体の構成要素に変換して 自己を維持し成長する過程のことです 生命は子孫に情報を伝えます ヒトは自分の情報を 遺伝子のDNAとして持ち これを子孫に伝えます 肉体と代謝という最初の二つの特徴を 合わせると 移動して複製もできる システムができます さらに情報の継承も取り込めば もっと生命に近いシステムとなります 進化もしていくかもしれません 実験室で我々が試みたのは 生命の特徴の1つか2つを 実験で示すことです
どうやったかというと プロトセルと呼ぶモデルを使いました ごく原始的な細胞を 想像してください 生きた細胞の化学モデルです 例えば皆さんの体の細胞では 百万種もの分子によって 複雑なネットワークが構成されて 「生きている」と呼べる 状態となります 実験室では同じようなことを 数10種類ほどの分子だけで 目指しています 複雑さは大幅に失われますが でも生命のように見える状態を作ろうとしています 私たちは簡単な実験から 高度化して 生きたシステムを目指します 100年前にルデュクが 人工生命について 残した言葉を見ていきましょう 「生命が合成されることがあっても 普通に考えられているような 驚くべき発見とはなるまい」 初めの一文です 実験室で生命を合成できたとしても 日常生活には何も影響しないでしょう
「進化論を受け入れれば 生命の合成の最初の きざしは無機と有機の 中間的な姿となるであろう すなわち非生命と生命の境目で 生命の原始的な特徴の 幾つかを有するだけの姿となろう」 先ほど述べた特徴ですね 「環境からの進化的なはたらきが 作用する中で少しずつ 他の特徴が加わるのだ」 まず単純な実験で 生命の特徴の一部を有するー 構造を作り そこから発展させて しだいに生命に近づけていきます プロトセルの試作はこのように始まります 自己集積化というアイデアを用います すなわち 試験管の中で 化学物質を混ぜると自然に 集まり始めて どんどん 大きな構造ができていくのです 数万個とか 数十万個もの分子が集まって 何もなかったところに 大きな構造を作ります この例では ある膜分子を利用しました 適切な環境で材料を混ぜると ものの数秒で こんなに複雑で美しい構造ができます これらの膜は 形態も機能も 生体の膜組織と大変似たものです そこでこの膜を使って 我々のプロトセルを作れるわけです
同様に 水と油のシステムも利用できます 油と水は互いに混ざりませんが 自己組織化によって きれいな油滴ができるので プロトセルとして人工生命体の 肉体にできます また後で お見せします ここまでは体の作り方でしたね 構造だけです 生体の他の側面はどうでしょうか 今お見せしているのも プロトセルですが 天然に産出される モンモリロナイトという粘土を使いました こんな粘土の塊が天然に得られます 化学的に活性な表面を形成し そこで代謝を起こすことができます この粘土にある種の分子がー 例えば 赤で示すRNAが集まります DNAと似ていて 情報を伝達する分子です RNAはこの粘土の表面に 集まってきます この構造はその周囲に 膜の境界を形成して 周りを液体分子で囲まれた 体を形成します 顕微鏡写真の緑の部分です 自己組織化によって 実験室で物質を混ぜてできた 膜の肉体の内側に 代謝を起こす表面があり 情報を持った分子が付いています
生体系へ向けた一歩です しかし このプロトセルを見て実際に 生きていると考えることはありません 生命のかけらも見当たらず 出来上がった後には 何もおきません 何かが足りません いくつかの点で足りていません 足りない点は 例えば この系を通るエネルギーの流れから エネルギーを少し取り込んで プロトセルが自らを 維持することができないことです 生体ならできることです さて別のプロトセルモデルを作りました 今のものよりも単純です このプロトセルではただの油滴が 内部で化学的代謝反応を起こし そのエネルギーで何かをするー プロトセルで このように 動いたりします この油を何滴か落とします 周りは水です プロトセルは動き始めます 自己組織化によってできた油滴の 内部では化学的代謝が行われ エネルギーを利用できます そのエネルギーを利用して 環境の中を動きます
前に述べたように 動くことは この種の生体系において重要です 動き回り 周囲の様子を探り 周囲を変化させます このように 化学的な波を起こしています 自己を保存しようとする生体のように 周囲に作用していると言えるのです この動くプロトセルを使って 別の実験をします 動いていますね ではここに食糧を加えてみます 青いものが見えます システムに食糧を与えます プロトセルが動き 食糧に遭遇して 自分で形を変えると 食糧の濃度の高いところに登っていき そこに留まります 体があるだけではなくて 代謝して エネルギーを利用して 動き回れるシステムです 周囲の環境を感知して 自らを維持するための 材料を見つけます
脳も神経系も持たない ただ袋詰めされた化学物質なのに こんな面白くて 生きものみたいに 複雑な振る舞いができるのです ここでは化学物質の種類は 皿を満たしている 水を含めても5種で こんな系ができました こんなプロトセルを 組み合わせる実験をすると 条件にもよりますが 左側では 動き回るプロトセルが そこに置かれた別のプロトセルと 接触しようとしています 右側では二つのプロトセルは ぐるぐる回るのが好きなようです とても複雑なダンスのようです この系で見たような プロトセル単体の 振る舞いだけではなく 集団レベルでの挙動もあり これも生体と似たものです みなさんもプロトセルの専門家になりましたので プロトセルでゲームをしてみましょう 二種類の違ったものを作ります プロトセルAはある種の化学物質を含み 活性化すると 振動して踊り始めます こんなに原始的だというのに 踊るプロトセルとは 興味深いですね (笑)
第二のプロトセルは別の化学物質を含み 活性化すると みんな集まってくっついて 一つの大きなプロトセルになります こんな二種類を 一緒にしてやるとどうなるか まずAを入れて Bも入れて そしてシステムを活性化すると 青い方のプロトセルBは 全部が集まって一つになり 大きな塊となります もう一種類はただ動いています 系のエネルギーがなくなるまで動いて それでおしまいです この実験を何度も繰り返すと あるとき とても面白いことが起きました 2つのプロトセルを系に加えると プロトセルのAとBが融合して ハイブリッドのABセルができました それまでなかったことです ここにはプロトセルABがあるのです プロトセルABはしばらく踊り続け プロトセルBが融合を続けます
そしてもっと面白いことがおきます 大きなハイブリッドのプロトセルが 融合するところを見てください しばらく踊った後 自己複製も起きました (笑) これまた化学物質の塊です この作用の仕方は 5種の化学物質だけの簡単なシステム 混成がおきるとき それまでとは違って もっと複雑なものができます また別の種類の生命と似た 特徴も現れました 複製をしたのです
こんなに面白いプロトセルは 色や挙動も魅力的で 作成するのも簡単で 生命のような特徴を持っています こんなプロトセルから地球の生命の 起源について学べることでしょう おそらく最初の段階では こんな簡単な姿から 地球上の生命は始まったことでしょう 実際 地球には初期から 分子が ありましたが この実験に使ったような 純粋な化合物では なかったでしょう むしろ 本当に複雑で あらゆる物が混ざり 制御されていない化学反応で生成した 多種多様な有機化合物の混ざった複合体 原始の泥を考えてください 水たまりに たまった茶色の タール状の生成物は 現代的な方法でも 完全には分析できません 右側に置いてある純粋な化合物と 比べてみてください
こちらは台所にある純粋な砂糖の 結晶を鍋に入れて加熱したときに 起きることと 似ています 砂糖に熱を加え 化学結合を加えたり切ったりして 茶色のカラメルが生成されます これを調整せずに続けると 化学結合の追加と切断が続き さらに多様な分子の混合体となって この種の黒いタール状のものが 鍋の中に残されます これは洗い流すのも大変です 生命の起源はこんなふうだったでしょう 45億年前の初期の地球では こんなどろどろの中から 生命は発生しなければなりませんでした 次の課題は 純粋な化学物質を離れて 生命のような性質を持ったプロトセルを この種の原始的な泥から作ることです
先ほどもお見せした 自己組織化した油滴の 登場です 内部の黒いしみは この種の黒くて多様で複雑な 有機物のタールです 先ほどお見せしたような実験に投入し そこから生命的な動きが 生じるかどうか観察します いい感じです すてきな動きです ある種の習性があるようで 互いに周りを回って 追っかけあっています 先ほど見せたのと似ています でも原始的な条件の下で行い 純粋な物質はありません これもタールで作ったプロトセルです 周囲から食糧を発見します 周囲から食糧を発見します 左側からエサを与えると ゆっくり広がってきます これは大好きなようで とても活発になって 周囲から食糧を見つけ出しました 前にお見せしたのとそっくりです これも原始の環境で実現しました 制御されていない条件です 小ぎれいな実験室条件ではありません 実際 とても汚い 小さなプロトセルです しかし 生体のような特徴のあることが重要です
こんな人工生命の実験をすることで 非生命と生命の間をつなぐ経路を 定義できるようになるかもしれません それだけでなく 生命とは何か そして生命の形態のー 可能性についての視野を拡げます 地上とは全く異なる 生命もあり得るのです そこから次の言葉が現れます 「奇妙な生命」 スティーブ・ベナールが名付けました 米国学術研究会議の 2007年の報告書に 登場しました 地球上とは大変違う形態の生命を 宇宙でどうやって探すべきかを 考察した内容です 別の惑星を訪れて そこに生命がいるだろうと考えたときに どうすれば生命だと判定できるでしょうか
三つの一般的な基準が示されています リストの一番です 第一に 非平衡の系でなければならない つまり 死滅したシステムではないこと つまり 死滅したシステムではないこと その基本的な意味は システムにエネルギーが与えられ それを利用して自己を保持しているということです 太陽が地球を照らし 光合成が行われて 生態系の原動力となるのと似ています 太陽がなかったら この星には生命は存在しないでしょう 第二に 生命は液体でなければならない つまり面白い構造だとしても 面白い分子であっても 動けない固体だと 生命には適しません 第三に 化学結合をしたり 切れたり しなければなりません 生命が環境から取り込んだ資源を 構成要素として取り込んで 自己を保持するために重要です
奇妙で不思議なプロトセルを紹介しました 粘土を含むもの 原始的な泥を含むもの 内部が水の代わりに ほぼ油だけで構成されたもの 大半はDNAを含んでいませんが 生命のような性質を示します これらのプロトセルは生命の 一般的な条件を満たします こんな化学的な人工生命の実験を通して 生命の起源と この惑星に生命が棲むことについて 基本的な理解が得られます さらにまた宇宙にはどんな生命の 可能性があるのかということも 明らかになります ありがとうございました (拍手)
品詞分類
- 主語
- 動詞
- 助動詞
- 準動詞
- 関係詞等
TED 日本語
TED Talks
関連動画
プラスチックを食べる細菌モーガン・ヴェイグ
2019.06.24微生物の世界へようこそ ― 自宅にも、そしてあなたの顔にもアン・マデン
2017.08.24CRISPRについて、みんなが知るべきことエレン・ヨルゲンセン
2016.10.24自然に潜む驚異的な力を生かす方法オーデッド・ショゼヨフ
2016.10.18自分でDNA検査ができる時代の到来セバスチャン・クレイベス
2016.10.13リンゴから耳を作るマッドサイエンティストアンドリュー・ペリング
2016.07.081つの生物種全体を永久に変えてしまう遺伝子編集技術ジェニファー・カーン
2016.06.02ゲノムを読んで人間を作る方法リッカルド・サバティーニ
2016.05.24宇宙での生存に備えて人類が進化する方法リサ・ニップ
2016.04.21干ばつに耐えられる農作物の作り方ジル・ファラント
2016.02.09DNA編集が可能な時代、使い方は慎重にジェニファー・ダウドナ
2015.11.12テクノロジーとバイオロジーを融合したデザインネリ・オックスマン
2015.10.29アニメーションで科学者の仮説を試す方法ジャネット・イワサ
2014.08.07二人の若き科学者、バクテリアでプラスチックを分解
2013.07.18毛長マンモスを復活させよう!ヘンドリック・ポイナー
2013.05.30私たちを取り巻く細菌と住環境のデザインジェシカ・グリーン
2013.03.25
洋楽 おすすめ
RECOMMENDS
洋楽歌詞
ダイナマイトビーティーエス
洋楽最新ヒット2020.08.20ディス・イズ・ミーグレイテスト・ショーマン・キャスト
洋楽人気動画2018.01.11グッド・ライフGイージー、ケラーニ
洋楽人気動画2017.01.27ホワット・ドゥ・ユー・ミーン?ジャスティン・ビーバー
洋楽人気動画2015.08.28ファイト・ソングレイチェル・プラッテン
洋楽人気動画2015.05.19ラヴ・ミー・ライク・ユー・ドゥエリー・ゴールディング
洋楽人気動画2015.01.22アップタウン・ファンクブルーノ・マーズ、マーク・ロンソン
洋楽人気動画2014.11.20ブレイク・フリーアリアナ・グランデ
洋楽人気動画2014.08.12ハッピーファレル・ウィリアムス
ポップス2014.01.08カウンティング・スターズワンリパブリック
ロック2013.05.31ア・サウザンド・イヤーズクリスティーナ・ペリー
洋楽人気動画2011.10.26ユー・レイズ・ミー・アップケルティック・ウーマン
洋楽人気動画2008.05.30ルーズ・ユアセルフエミネム
洋楽人気動画2008.02.21ドント・ノー・ホワイノラ・ジョーンズ
洋楽人気動画2008.02.15オンリー・タイムエンヤ
洋楽人気動画2007.10.03ミス・ア・シングエアロスミス
ロック2007.08.18タイム・トゥ・セイ・グッバイサラ・ブライトマン
洋楽人気動画2007.06.08シェイプ・オブ・マイ・ハートスティング
洋楽人気動画2007.03.18ウィ・アー・ザ・ワールド(U.S.A. フォー・アフリカ)マイケル・ジャクソン
洋楽人気動画2006.05.14ホテル・カリフォルニアイーグルス
ロック2005.07.06